Translate this page into:
Recovery of memory decline during aging - role of epigenetics
* Corresponding author: Dr. Mahendra Kumar Thakur, Department of Zoology, Institute of Science, Banaras Hindu University, Varanasi, India. Email: mktian2007@gmail.com
-
Received: ,
Accepted: ,
How to cite this article: Mishra E, Thakur MK. Recovery of memory decline during aging - role of epigenetics. Ann Natl Acad Med Sci (India). 2024;60:101–12. doi: 10.25259/ANAMS-2022-11-6-(777)
Abstract
Aging is a natural phenomenon associated with the accumulation of multiple alterations, including memory loss. Such deterioration of memory is based on the susceptibility of specific brain regions and the disorders that coincide with aging in those areas. Previous findings suggest that the optimal expression of synaptic plasticity-related genes is essential for memory formation and consolidation. Epigenetic modifications are one of the most crucial factors that cause memory deterioration by inducing the differential expression of synaptic plasticity-related genes. Understanding the fundamental cause of cognitive alterations that arise with aging is very essential for the development of therapeutic and/or preventive approaches. Several strategies have been employed to restore or reverse the memory decline caused by age-associated epigenetic alterations. The present article emphasizes the role of epigenetic alterations caused by histone modifications, DNA methylation, and non-coding ribonucleic acids (RNAs) on memory during aging. Also, we highlight the mechanistic switches of brain aging, including physical exercise, nutraceuticals, epigenetic modifiers, modulators of non-coding RNAs, and associated targets for therapeutic interventions. The emerging field of neuropharmacology and pharmacoepigenomics provides evidence that small drug molecules are currently employed to treat memory loss associated with aging, particularly by targeting epigenetic systems like DNA methylation, chromatin remodeling, histone modifications, and small non-coding RNAs. Therefore, targeting epigenetic modifications could be a potential therapeutic approach for the improvement of synaptic plasticity, neuronal activities, memory, and other brain functions during aging.
Keywords
Aging
Epigenetics
Memory
Pharmacoepigenomics
Therapeutic Recovery
INTRODUCTION
Memory-related issues are common complications in aged individuals and are considered as one of the prime concerns in even those who are healthy and do not have any neurological disorder.1,2 The changes in molecular, chemical, and physical characteristics of neurons with advancing age result in cognitive deficits, memory loss, dementia, and behavioral alterations.3 A key mechanism involved in the modification of neuronal networks and the formation of memory is attributed to the activation of neuronal plasticity-related genes. Age-associated cognitive decline is largely attributed to the progressive loss of synaptic plasticity caused by intracellular and intercellular alterations in the hippocampus.4 Studies on the effect of aging on memory have attracted attention due to the growing aged population.5 In several human subjects and animal models, the expression of synaptic plasticity-related genes is altered during the formation and/or loss of memory. The storage and duration of long-term memory occur at the cellular level as a result of gene transcription and translation. However, long-term memory declines with normal aging and in a variety of pathological conditions like dementia, neurodegenerative ailments, depression, and neuropsychiatric issues.6 Previous studies have reported alterations in the expression of various synaptic plasticity-related genes, including cAMP response element binding (CREB) protein, early growth response 1 (EGR1), glutamate receptor (GluR), activity-regulated cytoskeletal-associated (ARC) protein, and brain-derived neurotrophic factor (BDNF) during normal aging as well as pathological conditions.6–8 However, the molecular mechanism regulating the expression of synaptic plasticity-related genes is not elucidated. A study has demonstrated that these synaptic plasticity-related genes are significantly regulated at the transcriptional level by epigenetic mechanisms.9 Therefore, stable behavioral consequences, including the formation of long-lasting memory, may depend on the healthy function of the neuronal epigenome.
The epigenetic-driven changes in the transcriptome impair many of the brain processes with aging, including synaptic plasticity, learning, and memory.9 These impairments are caused by epigenetic alterations such as histone acetylation and methylation and DNA methylation, which regulate the expression of memory and synaptic plasticity-related genes. Several epigenetic mechanisms, including histone modifications, nucleosome remodeling, DNA methylation as well as non-coding RNAs (ncRNAs)-mediated gene regulation are known to alter with age.10 Considering the remarkable role of epigenetic modifications in the regulation of neuronal plasticity, several pharmacological antagonists and agonists are being used to improve cognitive functions by targeting these modifications that cause alterations in the transcription of memory-associated genes during aging.9 Thus, epigenome-targeted interventions are one of the most promising fields of anti-aging research because of the outstanding adaptability and flexibility of the neuroepigenome. In addition, various therapeutic approaches such as calorie restriction, antioxidant dietary supplements, lifestyle adjustments, brain-training exercises, and molecular targeting with epigenetic modifiers such as synthetic pharmacological compounds and natural bioactive compounds have been employed.11 The current review deals with memory and its regulation, the role of epigenetics in memory during aging and therapeutic approaches for memory recovery.
MEMORY AND ITS REGULATION DURING AGING
Memory is the ability to recite or recall information that has been learned and stored after any event.12 A crucial aspect of memory formation is attributed to neuroplasticity, which brings about long-lasting changes in the brain and behavior from brief stimulations.13 It has been widely accepted that the ability of an organism to adapt to a transient environmental stimulus over time is dependent on the capacity of the central nervous system for functional and structural plasticity.14 This plasticity depends on a meticulously controlled series of post-synaptic receptor activation, neurotransmitter release, intracellular signaling cascades, transcription of synaptic plasticity-related genes, and consequent protein synthesis. The comprehensive cellular and molecular alterations in the brain regions are responsible for acquisition, consolidation, and maintenance of long-term memories.9 The two key components, long-term depression (LTD) and long-term potentiation (LTP) are accountable for the neuroplasticity to induce prolonged changes in the brain and behavior.15 The ability to encode, store, and retrieve environmental information by the brain deteriorates with age under the influence of several physiological and epigenetic factors.16–19 Memory loss and the downregulation of synaptic plasticity-related genes are the consequences of several morphological, physiological, and molecular changes that take place in the brain with aging.20,21
Aging causes a delay in encoding, processing, and response time and has diverse effects on various types of memories.22 For instance, recognition memory, working memory, episodic memory, and prospective memory all significantly deteriorate with normal aging, whereas procedural memory and some perceptual memory skills exhibit negligible age-related changes.4 Although the capacity to recall extremely detailed information declines dramatically with age, semantic memory lasts well in an elderly person, if the information is used routinely.23 Free recall task tests revealed that older adults perform worse than younger adults, implying that to reach high levels of performance, advanced encoding and retrieval techniques are employed, which deteriorate with advancing age.24 During aging, deterioration in various cognitive functions including attention, episodic memory, and executive functions is dependent on the hippocampus and prefrontal cortex.25 The course of cognitive aging varies according to genetic and epigenetic factors that influence cellular damage and vulnerability or resistance to age-related stress.9 The limitation of the transient nature of memory-related biomarkers has been envisioned for the identification of more stable and adequate biomarkers with a longer duration even under the influence of several epigenetic factors. Therefore, an extensive investigation is required in the area of neuroepigenetics to understand the details of memory and its regulation during aging.
ROLE OF EPIGENETICS IN MEMORY
The covalent modifications of DNA and histone proteins can cause the conformational and structural alterations of chromatin, thereby upregulating or downregulating the transcription of associated genes and eventually leading to differential gene expression.26,27 Some enzymes and processes, such as DNA methylation and post-translational changes in histone proteins, in particular methylation, phosphorylation, ubiquitination, and acetylation, result in these alterations. During aging, the epigenetic dysregulation caused by DNA methylation and histone acetylation is responsible for memory impairment.13 Chromosomes are typically compressed, making it challenging for transcription factors to attach, and this leads to the suppression of memory-related genes. During chromatin relaxation, transcription factors bind easily to DNA and lead to the activation of genes related to memory. Therefore, chromatin expansion and contraction regulate the expression of various immediate early genes (IEGs) that are responsible for cognitive functions [Figure 1]. The epigenetic modifiers are known to upregulate the IEGs by inducing some of the specific transcription factors such as CREB and Elk1 which lead to the conversion of short-term memory into long-term memory.28,29 The IEGs such as Arc, BDNF, c-fos, CREB, and Zif268 are important factors responsible for LTD and LTP, and further, lead to the formation of long-term memory and its consolidation.29 Previous study revealed that the dysregulation in epigenetic regulatory mechanisms associated with the aging epigenome includes abnormally produced transcription factors, epigenetic modulators, enzymatic dysfunctions, and ncRNAs, which together cause a gradual loss of sensitivity to environmental cues and accelerate the aging process.27 Moreover, the impairments in the function and biogenesis of various ncRNAs cause initial and sustained synaptic loss and cognitive deficits in aging models.30 Further investigation in the field of neuroepigenetics is required to comprehend the underlying mechanisms of memory persistence.26 The subsequent sections deal with the involvement of various epigenetic factors that affect memory and its consolidation during aging.
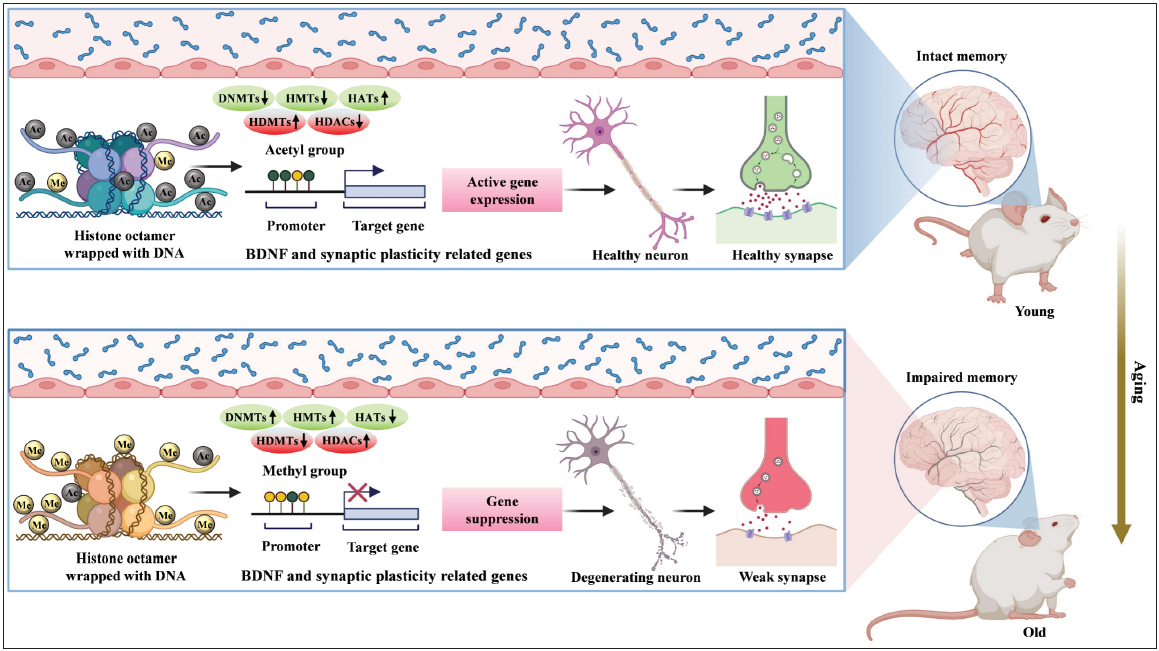
- Summary of age-related alterations in neuroepigenome driven by epigenetic modification of memory-related genes and proteins. In the young brain, the gene promoter is epigenetically modified by differential expression of chromatin-modifying enzymes (DNMTs, HMTs, HATs, HDMs, and HDACs). Increased expression of HATs and HDMTs, and decreased expression of DNMTs, HMTs, and HDACs results in the activation of genes related to cognitive functions and synaptic plasticity leading to intact memory in young. In contrast, the increased expression of HMTs, DNMTs, and HDACs, along with decreased expression of HATs and HDMTs is observed in old. These alterations result in the suppression of cognitive functions and synaptic plasticity-related genes leading to impaired memory in the old.
- DNMTs: DNA methyltransferases; HMTs: Histone methyltransferases; HATs: Histone acetyltransferases; HDMTs: Histone demethylases; HDACs: Histone deacetylases; BDNF: Brain-derived neurotrophic factor.
DNA METHYLATION IN MEMORY DURING AGING
DNA methylation can be dynamically changed throughout life, altering synaptic connectivity and cognitive functions with aging.10 During DNA methylation, a methyl group (CH3) is covalently attached to the 5’ site of cytosine residues in CpG dinucleotides. Penner et al. reported that aged rats show considerably higher levels of methylation at the Arc promoter than young rats.31 It has also been shown that a wide range of agonists of DNA methyltransferase (DNMT) activity modify DNA methylation status by binding to the promoter region of several neuronal plasticity-related genes such as Reelin, PP1, and BDNF. The promoter of the PP1 gene undergoes hypermethylation as a result of fear conditioning, which leads to the suppression of PP1 synthesis.32 On the other hand, Reelin supplementation in the CA1 region of the hippocampus enhances the memory formation, learning ability, and synaptic plasticity for an extended period. This suggests that reduction in Reelin signaling might cause diminished cognitive function in age-related neurodegenerative disorders.32 The hypomethylation at the promoter region of Reelin leads to the increased transcription of Reelin, which enhances the synaptic plasticity and cognitive functions.33 Another study revealed that the DNMT-knockout in vivo model exhibits deficiency in the Morris maze, contextual fear training, and hippocampal LTP.34,35 Furthermore, deficits in learning and LTP were demonstrated in an in vivo mice model having double knockout for DNMT3a and DNMT1 genes in neuronal cells of the forebrain but not in the single knockout of either DNMT3a or DNMT1 gene.34 This study suggests that the regulation of synaptic function and maintenance of DNA methylation status can be sustained by the presence of either of these isoforms. Memory formation involves both increased and decreased methylation at the promoter region of memory suppressor genes and memory enhancer genes, respectively. Therefore, either hypermethylation or hypomethylation might be responsible for memory function. These findings collectively imply that the regulation of DNMT activity is essential for memory and DNA methylation may cooperate with histone modifications, which have previously been linked to memory development and storage.35,34 Additionally, several studies have found abnormal DNA methylation patterns in many areas of the brain, notably at CpG and non-CpG sites, suggesting that aberrant DNA methylation potentially aids in neurodegeneration.36
HISTONE MODIFICATIONS IN MEMORY DURING AGING
Histone modifications alter the fundamental structure of chromatin by modifying histone tails post-translationally.10 A change in histone variants is associated with both gene activation and gene suppression. Acetylation of histones is linked to increased expression of genes, whereas deacetylation is linked to suppressed gene expression. In a study, the expression of histone deacetylase 2 (HDAC2) was elevated but there was no change in the level of CREB-binding protein (CBP) with HAT activity in the hippocampus of old mice.16 Furthermore, another study showed that the complex association of CREB with CBP leads to the inhibition of HDAC and thereby affects the long-term memory for novel object recognition in a mouse model.37 In the brain of aging mice, an increase in HDAC2 expression was linked to a worsening in recognition memory consolidation.38 Epigenetic instability has also been linked to the loss of memory in neurodegenerative diseases. For instance, after fear training, histone acetylation is decreased in the hippocampus of APP/PS1 mice, which may be attributed to an increased level of HDAC activity. This is supported by the finding that contextual fear conditioning and LTP deficiencies in aged APP/PS1 mouse models may be cured by inhibiting HDACs.39
The histone methyltransferases control both the epigenetic inheritance of genes and the integrity of the genome. Histone methylation is crucial to distinguish between the integrity of the genome and the genes which give identities to the cells. The addition of methyl groups to histones regulates transcription by blocking or enabling the transcription factors to bind to the promoter region of numerous IEGs and memory-associated genes. Moreover, transcriptional activation or repression of memory-associated genes depends on the position and the degree of histone methylation. It has been demonstrated that the pattern of histone methylation changes with aging, depending on the tissues and organisms. The expression level of H3K9me2 in the prefrontal cortex is higher in age-related neurodegenerative mice models, and the suppression of methyltransferases reversed deficiencies in spatial, working, and recognition memory.40 Among the H3K9me3-specific histone methyltransferases, SUV39H1 expression was noticeably elevated in aged mice. The global H3K9me3 level was also high in the hippocampus of aged mice. Old mice exhibited higher amounts of H3K9me3 at the IEGs promoter than young mice. The binding of H3K9me3 at the IEGs promoter further leads to a decline in recognition and spatial memory.41 The specific pharmacological antagonist is used to block SUV39H1, which result in a low level of hippocampal H3K9me3 and increases the level of BDNF protein and spine density in the aged mice model. These alterations lead to enhanced performance of behavioral tests, including fear conditioning, memory for object locations, and complicated spatial learning.42 Altogether, memory loss and its pathophysiology are largely attributed to the alteration in epigenetic pathways.
NON-CODING RNAS (NCRNAS) IN MEMORY DURING AGING
Non-coding RNAs can regulate the activity of genes by interacting with mRNAs, transcriptional stimulators, and other small RNAs and thereby restrict them to code for essential proteins related to memory.43 Aging and age-associated diseases are correlated with dysregulations in the biogenesis and functioning of ncRNAs. In functionally partitioned neurons, such regulation is crucial, which may explain the abundant biogenesis of potential ncRNAs in the brain.44 For optimal synaptic function, the pathways followed for the transportation of mRNAs to the synapse are essential, and their synaptic translation is managed in an activity-dependent way. In synaptic structures, a subset of conserved neuronal miRNAs is more prominent and involved in the regulation of transported transcripts associated with synaptic plasticity and memory.30 The upregulation of the evolutionarily conserved ncRNA, miR-132 is mediated by CREB and BDNF-dependent molecular pathways. The in vivo study showed the involvement of a neuronal activity regulating miR-132 in memory consolidation, learning, and synaptic plasticity in mice models. Further, a study revealed that in the hippocampus of transgenic mice, a comparatively lower expression level of miR-132 results in the enhancement of learning, novel object recognition capacity, and the induction of synaptic plasticity.45 These findings demonstrated that the function of neuronal cells, memory formation, learning, and synaptic plasticity are extremely sensitive to the concentration of miRNAs i.e., an increase in the expression level of miRNAs leads to neurodegeneration, which might be due to the inhibition of various essential mRNAs in an “off-target” way.46,47 The expression of three miRNAs such as miR-182, miR128b, and miR-134 is regulated during the formation of fear memories. The brain-specific miRNA, miR-134 regulates synaptic plasticity, learning, and memory formation by binding to the promoter region of BDNF and CREB.48 Furthermore, the deacetylase sirtuin 1 (SIRT1) regulates miR-134 in the brain, and its absence suppresses CREB and BDNF proteins, impairing learning and synaptic plasticity. Notably, the loss of miR-185 and miR-25 has shown age-dependent effects on synaptic plasticity in the hippocampus.44 In the coming future, it will be interesting to investigate the role of ncRNAs in controlling and maintaining the epigenetic factors associated with synaptic plasticity and memory formation in the aging brain.
THERAPEUTIC APPROACHES FOR THE RECOVERY OF MEMORY
Memory restoration involves the formation of new neurons and neuronal connections by providing additional resources and further re-establishes the structure and function of the neurons.48 Evidence from the emerging field of neuroepigenetics suggests that regulating the epigenetic mechanisms responsible for memory impairments could be a potential therapeutic strategy.49 An increasing line of evidence suggests that in memory loss conditions, the neuroepigenome can be altered by employing various strategies such as regular aerobic exercise, nutraceuticals, and pharmacological compounds to improve cognitive function.50 Memory is influenced by a variety of factors, some of which act as enhancers and others as inhibitors. In subsequent sections, we will be discussing some of the approaches that are involved in the recovery of memory.
PHYSICAL EXERCISE
Regular aerobic physical exercise helps in the maintenance of cognitive function during aging and prevents the onset of neurological diseases by altering the neuroepigenome.51 Intense exercise can promote DNA hypomethylation as it changes the methylation state of genes that respond to it. The capacity of neuronal cells to modify their form or to become more plastic depends on the position of methylation at CpG sites. Recent research in postnatal brain tissues has pinpointed the CpGs locations where physical training acutely modifies methylation state.10,52 The variations in the intensity of methylation at the CpG site caused by aerobic exercise affect the memory-associated molecular mechanisms. Changes in DNA methylation brought on by exercise may impact a variety of processes, including increased muscle contraction and other molecular alterations that are oxidative and non-oxidative, as well as the mitochondrial number. A previous study suggested that the downregulation of BDNF is observed in normal aging and age-related memory loss.53 The synthesis of neurotrophic factors such as BDNF is reported to be enhanced by physical exercise and is known to play a potential role in preserving neuronal health and memory. Moreover, physical exercise increases the expression level of BDNF by lowering the expression of HDAC.54 Running exercises have also been shown to upregulate the expression of hippocampal BDNF and synapsin-I, and thereby improving spatial memory. Exercise improves the ratio of acetylation to total protein for histone H3, but it does not affect histone H4. The HDAC5 enzyme, which is involved in regulating the expression of the BDNF gene, is likewise lowered by exercise at both protein and transcript levels.54 Previous studies show that physical exercise improves memory by inducing dendritic morphological alterations, such as an increase in dendritic arborization and dendritic spine density.51,55 Thus, regular exercise can help maintain intact memory by enhancing postsynaptic architecture and synaptic protein production, which can boost synaptic transmission and encourage synaptic plasticity.
NUTRACEUTICALS
The epigenome of an individual can be affected by the diet, which can alter gene expression and affect health.56 Epigenetic control and associated processes, including DNA methylation and one-carbon metabolism, are influenced by nutrition and dietary components, such as vitamin B12, folate, and methionine. To prevent or reverse the detrimental epigenetic alterations associated with aging, several dietary components have been discovered as epigenetic modifiers and used as an effective strategy to avoid memory loss.57–59 Different fruits and vegetables, such as apples, parsley, berries, onions, broccoli, and celery, contain a varying degree of polyphenols, including phenolic acids, flavonoids like anthocyanins or flavonols, and tannins. Various studies on in vivo models suggested that the administration of flavonoid-rich diets, such as ginkgo biloba, blueberries, and green tea, improve memory.60 The nutraceutical diets are believed to play a critical role in cognitive function due to their significant ability to scavenge free radicals, as well as their anti-inflammatory and neuroprotective properties,61 and their notable function as epigenetic modulators. Additionally, dietary substances can block the activity of DNMTs and thereby modify the methylation of related proteins.62,63 As a result, nutriepigenetic and nutriepigenomic molecules, which affect fundamental human health, have emerged as a potential area of research. For instance, the unique interaction between the environment and the genome is highlighted by polyphenols, especially at physiological doses. The administration of a high dose of polyphenols exhibits a substantial effect under the condition of restricted levels of methyl donors. S-Adenosylmethionine (SAM), the universal methyl donor for DNA and protein methyltransferases is produced in the methionine cycle from a variety of dietary precursors such as betaine, choline, folate, methionine, and vitamins, including B2, B6, and B12. Therefore, poor SAM production and widespread hypomethylation of DNA should occur when methyl donors are less readily available, and vice versa.63 The nutraceuticals are known to influence cerebral blood flow and synaptic plasticity-related changes and enhance the neuronal connection in the hippocampus, that alters memory processing.60 Fruits and vegetables with various nutraceutical properties and a healthy lifestyle offer an innovative therapeutic strategy for the prevention and treatment of memory-related problems. It was found that curcumin reduces the DNA hypermethylation at CpG sites present at the promoter region of peroxisome proliferator-activated receptor-alpha (PPAR-a). Additionally, ascorbic acid-induced differentiation and hypomethylation have been linked to a reduction in the expression of DNMT.58 Overall, these findings suggest that ascorbic acid may have the potential to act as a (co-) therapeutic agent for age-associated malfunction in memory as well as for reducing the inflammatory diseases linked to aging through DNA and histone demethylation.63 The benefits of dietary intervention in modulating the detrimental epigenetic modifications involved in memory loss during aging are now the subject of considerable investigation.
PHARMACOLOGICAL COMPOUNDS
The risk of acquiring a range of age-related complications may rise if the aging epigenome is changed or less sensitive to both preventive and curative epigenetic-modifying pharmacological compounds.64 Epigenetic modifiers can partially reverse the abnormal aging brought on by epigenetic drift.65 According to the genetic studies of children with neurodevelopmental problems, epigenetic modifiers that regulate DNA methylation and chromatin remodeling are necessary for brain development.66 Additionally, the epigenome changes have been linked to various neurodegenerative diseases including Alzheimer’s disease.67 The application of epigenetic modifiers including pharmacological compounds, enzyme inhibitors, nutraceuticals, physical exercise, and modulators of ncRNA alters the epigenetic modifications and thereby enhances memory consolidation [Figure 2].
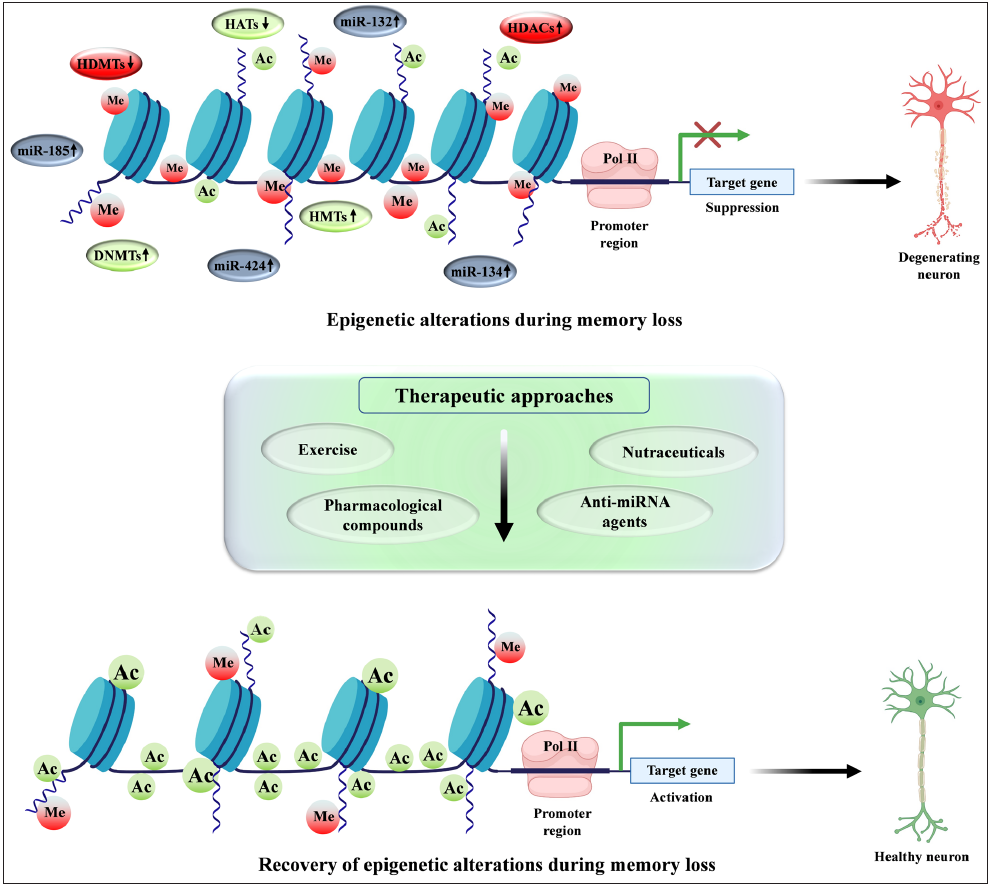
- A schematic model illustrating the recovery of memory decline using various therapeutic interventions. Increased methylation at the promoter region causes the suppression of various synaptic plasticity and memory-associated genes which lead to neurodegeneration and impaired memory. However, various therapeutic interventions including physical exercise, nutraceuticals, and pharmacological compounds work as memory enhancers and modulators. These therapeutic interventions lead to neuroprotection and memory restoration by following several biochemical pathways. These epigenetic modifiers cause increased acetylation and demethylation which in turn activates the transcription of various memory-related genes which leads to neuroprotection and thereby enhances synaptic plasticity and memory. These memory enhancers, including epigenetic modifiers, could potentially serve as ideal therapeutic targets in neuroprotection and memory loss conditions. HDMTs: Histone demethylases; DNMTs: DNA methyltransferases; HMTs: Histone methyltransferases; HATs: Histone acetyltransferases; HDACs: Histone deacetylases; Pol II: Polymerase II; miRNA: MicroRNA; Ac: Acetylation; Me: Methylation.
In light of recent advances in drug discovery and development, a variety of natural and synthetic bioactive compounds with pharmacological significance have been discovered to target epigenetic alterations, in particular, histone modifications and DNA methylation [Tables 1a and 1b]. Donepezil, Verapamil, Agmatine, Suvorexant, Memantine, Galantamine, and Rivastigmine have shown significant pharmacological activities in terms of cognitive improvement, responders’ rate, and dropout cases during aging.68 Donepezil promotes the activation of various genes by increasing the acetylation through decreased expression of HDAC2, and decreasing the histone methylation H3K9me2.69 Furthermore, the hypermethylation in the CpG island of the promoter region of muscarinic receptor type 3 may reduce the recognition memory in both humans and animals. Consequently, several muscarinic receptor antagonists work by altering the histone modification and DNA methylation status, thereby improving synaptic plasticity and memory.70,71
(a) Natural pharmacological compounds, their targets and role | ||
---|---|---|
Natural pharmacological compounds | Target | Role of the compound |
Spermidine | HATs | Significantly affects cognitive function |
Garcinol | HATs | Anti-inflammatory and antioxidant properties |
Spermine | HATs | Improves memory impairment via BDNF and TrkB activation |
Curcumin | HATs | Antioxidant and cognitive enhancer |
Epigallocatechin gallate |
HDACs | Lowers inflammation, stimulates endogenous antioxidant defense, and has an anti-aging skin impact |
Folate | HMTs | Deduces oxidative stress and keeps neurons intact during aging, all of which contribute to improved memory |
Vitamin B12 | HMTs | Improves neurocognitive problems |
Catechins | DNMTs | Inhibits age-related cognitive loss by increasing the expression of IEGs that are engaged in long-term changes in the plasticity of synapses and brain circuits |
Caffeic acid | DNMTs | Enhances hippocampal neurogenesis and memory |
Resveratrol |
DNMTs, HATs, HDACs, SIRT1 |
Beneficial effect on memory and aging |
S-Adenosylmethionine | HMTs | PSEN1 repressor and memory enhancer |
Nicotinamide | SIRT | Inhibits gene silencing and promotes replicative aging |
(b) Synthetic pharmacological compounds, their targets and role | ||
---|---|---|
Synthetic pharmacological compounds | Target | Role of the compound |
5-Azacytidine | DNMTs | Restores cognition in sevoflurane-exposed rats with cognitive impairment, object recognition memory in scopolamine-induced amnesic mice, and recognition memory in ovariectomized mice. |
ETP69 | HMTs | Gives the aging brain procognitive benefits, and this effect is proof that H3K9me3 is involved in memory function. It boosts the quantity of both stubby and thin spines required for the formation of new synapses, which makes them excellent for fostering rapid cognitive advances. |
BIX-01294 | HMTs | Restores the consolidation of fear memory. |
Tranylcypromine | HMTs | Prevents the death of dopaminergic neurons and restores the memory. |
Entinostat (MS-275) | HDACs | It enhances social connection and serves as an antipsychotic in a mouse model of social defeat. |
Suberoylanilide hydroxamic acid (SAHA) |
HDACs | Restores spatial memory in mice with impaired memory caused by sevoflurane as well as fear memory in CBP mutant mice and APP/PS1 AD mice. Improves mice’s recollection of their fears. Improvements in social interaction and antidepressant-like effects in a mouse model of social defeat. |
Trichostatin-A (TSA) | HDACs | Restores memory for identification in ovariectomized mice, and rescues memory for fear in CBP mutant mice. enhances memory for object identification in a mouse model of neurodegeneration treated with kainic acid, and improves the HD mouse model’s long-term memory impairment. |
Sodium phenylbutyrate | HDACs | Increases neurotrophin levels in the CNS and thereby enhances synapse function. Memory improvement, Amyloid burden reduction. |
Valproic acid | HDACs | Improves working memory difficulties in the hippocampal region and neurogenesis. Mood stabilizer, GABA modulator. |
Sodium valproate | HDACs | Reduces the defects in synaptic plasticity, ameliorates hippocampal neurodegeneration, and improves spatial memory. |
Vorinostat | HDACs | Memory improvement |
Sodium butyrate | HDACs | Restores object localization, spatial, and contextual fear memories in CBP mutant mice, fear memory in APP/PS1 AD mice, and fear memory in scopolamine-induced amnesic mice. promotes long-term memory retention while maintaining short-term memory function, improves fear memory and object recognition memory in elderly mice, and enhances recognition memory in cognitively deficient, maternally deprived rats. enhances both short-term and long-term memory for objects in a mouse model of neurodegenerative disease caused by kainic acid. Improves the behavior of mice with depression. |
Donepezil | HDACs, HMTs | Prevents neurodegeneration and memory improvement. |
Zebularine | DNMTs | Regulates synaptic plasticity, LTP induction, and learning and memory functions in the hippocampus. |
HATs: Histone acetyltransferases; HDACs: Histone deacetylases; DNMTs: DNA methyltransferases; SIRT: Silent Mating-Type Information Regulation; HMTs: Histone methyltransferases; BDNF: Brain-Derived Neurotrophic Factor; TrkB: Tropomyosin receptor kinase B; IEGs: Immediate Early Genes; PSEN1: Presenilin 1; CBP: CREB-binding protein; APP/PS1: Amyloid precursor protein/presenilin-1; HD: Huntington’s disease; CNS: Central nervous system; LTP: Long-term potentiation; GABA: Gamma-aminobutyric acid.
Since DNMTs are primarily known as negative regulators of memory, their inactivation could be targeted to restore memory during aging.71 The two most used commercial chemicals are 5-Azacytidine (5-Aza) and Zebularine available as DNMT inhibitors and function similarly to cytosine. These commercially available chemicals are DNA-binding agents that bind to DNA during replication and prevent the covalent binding of DNMTs, which results in demethylation and gene reactivation.63 The administration of pharmacological inhibitors of DNMTs such as 5-Aza and Zebularine reduces the methylation state of reelin and shows the region-specific impact of DNMT inhibition on the promoter of BDNF, which improves the synaptic plasticity. The incubation of rat hippocampus slices with the methylation inhibitor 5-Aza exhibits decreased methylation of the BDNF gene than that of the untreated slices.72 Administration of DNMT inhibitors 5-Aza, Zebularine, and RG-108 into the anterior cingulated cortical area reduces methylation of calcineurin gene and thereby impacts long-term memory.72 Zhao et al. demonstrated that intrahippocampal infusion of 5-Aza followed by behavioral training enhances the consolidation of recognition memory.73
Trichostatin A (TSA) and sodium butyrate (NaB) inhibit different classes of HDACs.73 In a mouse model of neurodegenerative disease, the non-specific HDAC inhibitor NaB dramatically improves the consolidation of spatial and associative memories.74 In agreement with the previous findings, intrahippocampal injection of NaB improves the acetylation level of H4K12 in the promoter region of the synaptic plasticity-related gene and associated fear-conditioned memory. The administration of NaB in the hippocampus of CBP mutant mice exhibits the restoration of object location memory consolidation.75 Also, post-training treatment of NaB in aging rats leads to increased levels of histone H4K8 acetylation in the promoter region of the synaptic plasticity gene BDNF than that of the control. Additionally, in scopolamine-induced amnesic mice, NaB therapy enhances memory consolidation by increasing the acetylation of total histone H3K9 and H3K14 as well as the promoter region of the synaptic plasticity genes such as BDNF and Arc.75 To improve memory and learning, suberoylanilide hydroxamic acid (SAHA) is also extensively employed as an HDAC inhibitor. In CBP mutant mice, intraventricular injection of SAHA improves the consolidation of fear memories and raises histone H2B acetylation levels and late-phase LTP. The proteome-based study revealed that HDAC1 and HDAC2 were identified as major targets of the SAHA-based affinity probe, suggesting that they may be pertinent targets for HDAC inhibition-induced memory improvement.76 Further studies suggested that intraperitoneal administration of SAHA improved the consolidation of fear memories in HDAC2 overexpressing mice.77 Besides the pharmacological compounds, several ncRNAs are also targeted for the restoration of memory.67 MicroRNAs have the potential to play a significant role in the processes that underlie memory loss brought on by aging and neurodegeneration. In the in vivo epilepsy model, the administration of miR-134 antagonists or miR-22 mimic-miRs was able to diminish neuronal loss and seizure intensity. The efficacy of administering anti-miRNA agents demonstrates an acceptable level of treatment tolerability, suggesting their potential utility for therapeutic interventions, particularly in the context of neurological disorders.46,78 These findings collectively imply that modifications in the gene-specific targeting of histone modifications may affect memory across the lifetime.
CONCLUSION
Memory acquisition and preservation are intricate processes that must be deliberately regulated for normal day-to-day activity. The ever-improving understanding of the physiology of memory is crucial for the development of novel therapeutic approaches to mitigate memory-related problems. Various epigenetic modifiers and their upstream molecules are responsible for the differential expression of genes and proteins related to synaptic plasticity and memory impairment or consolidation during aging. Moreover, memory impairment is potentially driven by the disruption of chromatin markers that lead to significant drift in neuroepigenome over time during aging. However, the detailed molecular mechanism causing alteration in these epigenetic markers during aging needs to be elucidated. Despite considerable research, efforts aimed at targeting the epigenetic markers for memory restoration during aging and various neurological disorders, several unresolved queries still need to be addressed. (A) How is aging correlated with the accumulation of epigenetic alterations in diverse types of epigenomic signaling involved in memory, and how do these modifications crosstalk? (B) What are the underlying mechanisms involved in the neuroepigenome of a stable or transient model of memory impairment following exercise and administration of the pharmacologic agents? (C) What is the significance of investigating neuroepigenetics to understand memory and its regulation during aging, considering the limitation of transient memory-related biomarkers and the need for more stable and long-lasting biomarkers that can withstand the influence of various epigenetic factors? (D) How do nutriepigenetic and nutriepigenomic molecules modulate epigenetic alterations and improve learning and memory? (E) What is the mechanism by which dietary intervention mitigates the adverse impact of epigenetic modifications linked to memory impairment in the aging process?
Several recovery approaches have been utilized to mitigate the problems associated with memory impairment caused by epigenetic alterations. Moreover, in-depth research is needed for the development of improved detection methods and the discovery of pharmacological drugs, including epigenetic modifiers to gain a complete understanding of the molecular pathways involved in memory. The understanding of epigenetic modifiers that control cognition and cause the reversal of age-related cognitive deficits will be a better therapeutic approach in the future. The implementation of innovative epigenetic-based prevention and therapeutic techniques will be helpful in diminishing memory-related problems. In addition to pharmacological compounds, physical exercise and numerous dietary components that alter neuroepigenome have also been found to delay aging and ward off associated problems. Further research is required to ascertain the ideal dose and time window for a range of dietary components and synthetic compounds, to effectively cure detrimental epigenetic effects against aging to improve the overall quality of life.
Acknowledgments
We acknowledge the Council of Scientific & Industrial Research (CSIR) for the Junior and Senior Research Fellowship and Indian Council of Medical Research (ICMR), New Delhi, for the Senior Research Fellowship to EM.
Authors’ contributions
EM: Conceptualization, Software, Visualization, Writing–original draft, Writing – review & editing; MKT: Conceptualization, Funding acquisition, Project administration, Resources, Supervision, Writing – final review & editing.
Ethical approval
Institutional Review Board approval is not required.
Declaration of patient consent
Patient consent is not required as the patients’ identity is not disclosed or compromised.
Financial support and sponsorship
Financial support was provided through research grants from ICMR (5/4–5/153/Neuro/2015-NCD-I), Department of Science & Technology (EMR/2015/002178), Government of India, and National Academy of Sciences, India-Senior Scientist fellowship (NASI-303/12/2021) to MKT.
Conflicts of interest
The authors declare that they have no real or potential conflicts of interest in terms of their personal, intellectual or financial interests.
Use of artificial intelligence (AI)-assisted technology for manuscript preparation
The authors confirm that there was no use of artificial intelligence (AI)-assisted technology for assisting in the writing or editing of the manuscript and no images were manipulated using AI.
References
- Ageing, neurodegeneration and brain rejuvenation. Nature. 2016;539:180-86.
- [CrossRef] [PubMed] [PubMed Central] [Google Scholar]
- Memory rehabilitation: Restorative, specific knowledge acquisition, compensatory, and holistic approaches. Cogn Process. 2022;23:537-57.
- [CrossRef] [PubMed] [PubMed Central] [Google Scholar]
- Mitochondria and aging: A role for the mitochondrial transition pore? Aging Cell. 2018;17:e12793.
- [CrossRef] [PubMed] [PubMed Central] [Google Scholar]
- Alterations in hippocampal mitochondrial dynamics are associated with neurodegeneration and recognition memory decline in old male mice. Biogerontology. 2022;23:251-71.
- [CrossRef] [PubMed] [PubMed Central] [Google Scholar]
- Alzheimer’s and non-Alzheimer’s dementia: A critical review of pharmacological and nonpharmacological strategies. Am J Alzheimers Dis Other Demen. 2008;23:150-61.
- [CrossRef] [PubMed] [PubMed Central] [Google Scholar]
- Young CSF restores oligodendrogenesis and memory in aged mice via fgf17. Nature. 2022;605:509-15.
- [CrossRef] [PubMed] [PubMed Central] [Google Scholar]
- Theories of memory and aging: A look at the past and a glimpse of the future. J Gerontol B. 2017;72:82-90.
- [CrossRef] [PubMed] [PubMed Central] [Google Scholar]
- Mitophagy: A promising therapeutic target for neuroprotection during ageing and age‐related diseases. Br J Pharmacol. 2023;180:1542-61.
- [CrossRef] [PubMed] [Google Scholar]
- Epigenetic mechanisms of learning and memory: Implications for aging. Int J Mol Sci. 2020;21:6918.
- [CrossRef] [PubMed] [PubMed Central] [Google Scholar]
- Epigenetic mechanisms related to cognitive decline during aging. J Neurosci Res. 2020;98:234-46.
- [CrossRef] [PubMed] [Google Scholar]
- Recovery of age-related memory loss: Hopes and challenges. In: Prasad S, Rath PC, Sharma R, eds. Topics in biomedical gerontology. New York: Springer Press; 2017. p. :267-78.
- [Google Scholar]
- Epigenetic regulation and chromatin remodeling in learning and memory. Exp Mol Med. 2017;49:e281.
- [CrossRef] [PubMed] [PubMed Central] [Google Scholar]
- Mdivi-1 rescues memory decline in scopolamine-induced amnesic male mice by ameliorating mitochondrial dynamics and hippocampal plasticity. Mol Neurobiol. 2023;60:5426-49.
- [CrossRef] [PubMed] [PubMed Central] [Google Scholar]
- Epigenetic regulation of memory formation and maintenance. Learn Mem. 2013;20:61-74.
- [CrossRef] [PubMed] [PubMed Central] [Google Scholar]
- Molecular and cellular aspects of age-related cognitive decline and Alzheimer’s disease. Behav Brain Res. 2017;322:191-205.
- [CrossRef] [PubMed] [PubMed Central] [Google Scholar]
- Reduced recognition memory is correlated with decrease in DNA methyltransferase1 and increase in histone deacetylase2 protein expression in old male mice. Biogerontology. 2014;15:339-346.
- [CrossRef] [PubMed] [Google Scholar]
- Altered histone acetylation is associated with age-dependent memory impairment in mice. Science. 2010;328:753-6.
- [CrossRef] [PubMed] [Google Scholar]
- The influence of colour on memory performance: A review. Malays J Med Sci. 2013;20:3.
- [PubMed] [PubMed Central] [Google Scholar]
- Posttraining systemic administration of the histone deacetylase inhibitor sodium butyrate ameliorates aging-related memory decline in rats. Behav Brain Res. 2011;221:329-32.
- [CrossRef] [PubMed] [PubMed Central] [Google Scholar]
- Hippocampal chromatin‐modifying enzymes are pivotal for scopolamine‐induced synaptic plasticity gene expression changes and memory impairment. J Neurochem. 2015;134:642-51.
- [CrossRef] [PubMed] [Google Scholar]
- Ageing and the brain. Postgrad Med J. 2006;82:84-88.
- [CrossRef] [PubMed] [PubMed Central] [Google Scholar]
- Memory abilities during normal aging. Int J Neurosci. 1998;93:151-62.
- [CrossRef] [PubMed] [Google Scholar]
- Aging and memory: A cognitive approach. Can J Psychiatry. 2008;53:346-53.
- [CrossRef] [PubMed] [Google Scholar]
- Aging and recognition memory: A meta-analysis. Psychol Bull. 2019;145:339-71.
- [CrossRef] [PubMed] [PubMed Central] [Google Scholar]
- Aging in The brain: New roles of epigenetics in cognitive decline. Neuroscientist. 2018;24:516-25.
- [CrossRef] [PubMed] [Google Scholar]
- The contribution of epigenetic inheritance processes on age-related cognitive decline and Alzheimer’s disease. Epigenomes. 2021;5:15.
- [CrossRef] [PubMed] [PubMed Central] [Google Scholar]
- Epigenetic alterations in Alzheimer’s disease. Front Behav Neurosci. 2015;9:347.
- [CrossRef] [PubMed] [PubMed Central] [Google Scholar]
- Transcriptional co‐repressor sin 3a silencing rescues decline in memory consolidation during scopolamine‐induced amnesia. J Neurochem. 2018;145:204-16.
- [CrossRef] [PubMed] [Google Scholar]
- Role of immediate-early genes in synaptic plasticity and neuronal ensembles underlying the memory trace. Front Mol Neurosci. 2016;8:78.
- [CrossRef] [PubMed] [PubMed Central] [Google Scholar]
- Memory decline and its reversal in aging and neurodegeneration involve mir-183/96/182 biogenesis. Mol Neurobiol. 2019;56:3451-62.
- [CrossRef] [PubMed] [Google Scholar]
- Age-related changes in arc transcription and dna methylation within the hippocampus. Neurobiol Aging. 2011;32:2198-210.
- [CrossRef] [PubMed] [PubMed Central] [Google Scholar]
- Reelin and apoe receptors cooperate to enhance hippocampal synaptic plasticity and learning. J Biol Chem. 2002;277:39944-52.
- [CrossRef] [PubMed] [Google Scholar]
- Role of epigenetics in age-related long-term memory loss, epigenetics of aging. New York, NY: Springer; 2010. p. :275-81.
- Impact of DNMT1 and DNMT3a Forebrain knockout on depressive and anxiety like behavior in mice. Neurobiol Learn Memory. 2016;135:139-45.
- [CrossRef] [PubMed] [PubMed Central] [Google Scholar]
- DNMT1 and DNMT3a maintain DNA methylation and regulate synaptic function in adult forebrain neurons. Nat Neurosci. 2010;13:423-30.
- [CrossRef] [PubMed] [PubMed Central] [Google Scholar]
- CpG and non-CpG presenilin1 methylation pattern in course of neurodevelopment and neurodegeneration is associated with gene expression in human and murine brain. Epigenetics. 2020;15:781-99.
- [CrossRef] [PubMed] [PubMed Central] [Google Scholar]
- HDAC inhibition modulates hippocampus-dependent long-term memory for object location in a CBP-dependent manner. Learn Memory. 2011;18:71-9.
- [CrossRef] [PubMed] [PubMed Central] [Google Scholar]
- Histone deacetylase 2 inhibition attenuates downregulation of hippocampal plasticity gene expression during aging. Mol Neurobiol. 2018;55:2432-42.
- [CrossRef] [PubMed] [PubMed Central] [Google Scholar]
- Dysregulation of histone acetylation in the App/Ps1 mouse model of Alzheimer’s disease. J Alzheimers Dis. 2009;18:131-9.
- [CrossRef] [PubMed] [PubMed Central] [Google Scholar]
- H3K9me2 regulation of BDNF expression in the hippocampus and medial prefrontal cortex is involved in the depressive-like phenotype induced by maternal separation in male rats. Psychopharmacol. 2021;238:2801-13.
- [CrossRef] [PubMed] [Google Scholar]
- Increase in hippocampal histone H3k9me3 is negatively correlated with memory in old male mice. Biogerontol. 2020;21:175-89.
- [CrossRef] [PubMed] [Google Scholar]
- H3K9me3 inhibition improves memory, promotes spine formation, and increases bdnf levels in the aged hippocampus. J Neurosci. 2016;36:3611-22.
- [CrossRef] [PubMed] [PubMed Central] [Google Scholar]
- Non-coding RNAs, epigenetic memory and the passage of information to progeny. RNA Biol. 2009;6:242-7.
- [CrossRef] [PubMed] [PubMed Central] [Google Scholar]
- Non-coding RNA regulation of synaptic plasticity and memory: Implications for aging. Ageing Res Rev. 2014;17:34-42.
- [CrossRef] [PubMed] [PubMed Central] [Google Scholar]
- MiRNA-132: A dynamic regulator of cognitive capacity. Brain Struct Funct. 2013;218:817-31.
- [CrossRef] [PubMed] [PubMed Central] [Google Scholar]
- Advances in roles of miR-132 in the nervous system. Front Pharmacol. 2017;8:770.
- [CrossRef] [PubMed] [PubMed Central] [Google Scholar]
- An activity-regulated microrna controls dendritic plasticity by down-regulating p250GAP. Proc Natl Acad Sci USA. 2008;105:9093-8.
- [CrossRef] [PubMed] [PubMed Central] [Google Scholar]
- Neuroregeneration and plasticity: A review of the physiological mechanisms for achieving functional recovery postinjury. Mil Med Res. 2020;7:30-45.
- [CrossRef] [PubMed] [PubMed Central] [Google Scholar]
- Epigenetics in neuronal regeneration. Semin Cell Dev Biol. 2020;97:63-73.
- [CrossRef] [PubMed] [PubMed Central] [Google Scholar]
- Epigenetic enzymes: A role in aging and prospects for pharmacological targeting. Ageing Res Rev. 2021;67:101312.
- [CrossRef] [PubMed] [Google Scholar]
- Neuroprotective effects of physical activity on the brain: A closer look at trophic factor signaling. Front Cell Neurosci. 2014;8:170.
- [CrossRef] [PubMed] [PubMed Central] [Google Scholar]
- Aerobic exercise training-induced changes on dna methylation in mild cognitively impaired elderly African Americans: Gene, exercise, and memory study-GEMS-I. Front Mol Neurosci. 2022;14:752403.
- [CrossRef] [PubMed] [PubMed Central] [Google Scholar]
- Physical exercise and health: A focus on its protective role in neurodegenerative diseases. J Funct Morphol Kinesiol. 2022;7:38.
- [CrossRef] [PubMed] [PubMed Central] [Google Scholar]
- Exercise impacts brain‐derived neurotrophic factor plasticity by engaging mechanisms of epigenetic regulation. Eur J Neurosci. 2011;33:383-90.
- [CrossRef] [PubMed] [PubMed Central] [Google Scholar]
- Effects of exercise intensity on spatial memory performance and hippocampal synaptic plasticity in transient brain ischemic rats. PLoS ONE. 2013;8:e78163.
- [CrossRef] [PubMed] [PubMed Central] [Google Scholar]
- Role of epigenetics in pharmacotherapy, psychotherapy and nutritional management of mental disorders. J Clin Pharm Ther. 2012;37:499-501.
- [CrossRef] [PubMed] [Google Scholar]
- The role of nutraceuticals as a complementary therapy against various neurodegenerative diseases: A mini-review. J Tradit Complement Med. 2020;10:434-9.
- [CrossRef] [PubMed] [PubMed Central] [Google Scholar]
- Nutrition and nutraceuticals in neuroinflammatory and brain metabolic stress: Implications for neurodegenerative disorders. CNS Neurol Disord Drug Targets. 2018;17:680-8.
- [CrossRef] [PubMed] [Google Scholar]
- Age-related epigenetic drift and phenotypic plasticity loss: Implications in prevention of age-related human diseases. Epigenomics. 2016;8:1637-51.
- [CrossRef] [PubMed] [PubMed Central] [Google Scholar]
- Effects of fruit and vegetable-based nutraceutical on cognitive function in a healthy population: Placebo-controlled, double-blind, and randomized clinical trial. Antioxidants. 2021;10:116.
- [CrossRef] [PubMed] [PubMed Central] [Google Scholar]
- Vitamin B12-folic acid supplementation improves memory by altering mitochondrial dynamics, dendritic arborization, and neurodegeneration in old and amnesic male mice. J Nutr Biochem. 2024;124:109536.
- [CrossRef] [PubMed] [Google Scholar]
- Effects of Ginkgo Biloba Extract (EGb 761) on learning and possible actions on aging. J Physiol Paris. 1997;91:291-300.
- [CrossRef] [PubMed] [Google Scholar]
- Epigenetic modulation of DNA methylation by nutrition and its mechanisms in animals. Anim Nutr. 2015;01:144-51.
- [CrossRef] [PubMed] [PubMed Central] [Google Scholar]
- Pharmacological enhancement of memory or cognition in normal subjects. Front Syst Neurosci. 2014;8:90.
- [CrossRef] [PubMed] [PubMed Central] [Google Scholar]
- Epigenetic modifiers: Basic understanding and clinical development. Clin Cancer Res. 2009;15:3918-26.
- [CrossRef] [PubMed] [PubMed Central] [Google Scholar]
- Epigenetics modifiers: Potential hub for understanding and treating neurodevelopmental disorders from hypoxic injury. J Neurodev Disord. 2020;12:1-12.
- [CrossRef] [PubMed] [PubMed Central] [Google Scholar]
- Epigenetic mechanisms in memory and cognitive decline associated with aging and Alzheimer’s disease. Int J Mol Sci. 2021;22:12280.
- [CrossRef] [PubMed] [PubMed Central] [Google Scholar]
- Donepezil in Alzheimer’s disease: From conventional trials to pharmacogenetics. Neuropsychiatr Dis Treat. 2007;3:303-33.
- [CrossRef] [PubMed] [PubMed Central] [Google Scholar]
- Changes in neuroimmune and neuronal death markers after adolescent alcohol exposure in rats are reversed by Donepezil. Sci Rep. 2019;9:1-12.
- [CrossRef] [PubMed] [PubMed Central] [Google Scholar]
- Epigenetic modulation of the muscarinic type 3 receptor in salivary epithelial cells. Lab Invest. 2015;95:237-45.
- [CrossRef] [PubMed] [Google Scholar]
- Epigenetics and pharmacoepigenetics of age-related neurodegenerative disorders. In: Cacabelos R, ed. Pharmacoepigenetics. Oxford, UK: Elsevier; 2019. p. :903-50.
- [Google Scholar]
- Inhibition of DNA methylation impairs synaptic plasticity during an early time window in rats. Neural Plast. 2016;2016:4783836.
- [CrossRef] [PubMed] [PubMed Central] [Google Scholar]
- Epigenetic alterations regulate the estradiol-induced enhancement of memory consolidation. Proc Natl Acad Sci USA. 2010;107:5605-10.
- [CrossRef] [PubMed] [PubMed Central] [Google Scholar]
- Enhancement of memory consolidation by the histone deacetylase inhibitor sodium butyrate in aged rats. Neurosci Lett. 2015;594:76-81.
- [CrossRef] [PubMed] [Google Scholar]
- Hippocampal focal knockout of CBP affects specific histone modifications, long-term potentiation, and long-term memory. Neuropsychopharmacol. 2011;36:1545-56.
- [CrossRef] [PubMed] [PubMed Central] [Google Scholar]
- Activity-based probes for proteomic profiling of histone deacetylase complexes. Proc Natl Acad Sci USA. 2007;104:1171-6.
- [CrossRef] [PubMed] [PubMed Central] [Google Scholar]
- HDAC2 negatively regulates memory formation and synaptic plasticity. Nature. 2009;459:55-60.
- [CrossRef] [PubMed] [PubMed Central] [Google Scholar]
- Addressing alzheimer’s disease (AD) neuropathology using anti-microRNA (AM) strategies. Mol Neurobiol. 2019;56:8101-8108.
- [CrossRef] [PubMed] [PubMed Central] [Google Scholar]