Translate this page into:
DPI: A paradigm shift from nebulizers to dry powder inhalers
*Corresponding author: Mr. Pradeep Shamgouda Patil, M. Pharm, Department of Pharmaceutics, R.C. Patel Institute of Pharmaceutical Education and Research, Karwand Naka, Shirpur, Maharashtra, India. pradeepspatil19@gmail.com
-
Received: ,
Accepted: ,
How to cite this article: Patil PS, Mahajan HS. DPI: A paradigm shift from nebulizers to dry powder inhalers. Ann Natl Acad Med Sci (India) 2024;60:250-60. doi: 10.25259/ANAMS_45_2024
Abstract
Drug delivery to pulmonary routes was once considered a challenging task. The emergence of inhalation therapy paved the way for drug delivery to the lungs. With various advances in inhalation therapy, modern-age drug delivery systems include nebulizers, pressurized metereddose inhalers, and dry powder inhalers (DPIs). The following review discusses the development stages of DPIs and also gives an insight into the current formulation aspects of DPI, animal models used for evaluation of drug release parameters, and the Anderson cascade impactor and its possible correlation with human respiratory tract with regards to drug deposition and particle size. Today, DPIs have brought about a revolution in drug delivery to lungs, and also offer more advantages with regards to consistency of dose delivered, stability, and ease of administration. Dry powders have been extensively used in the treatment of asthma and various other respiratory disorders. With the introduction of nanoformulations, a wide sector of researchers is working toward developing DPIs that may be used to target diseases like lung cancer.
Keywords
Aerodynamic diameter
dry powder inhalers
preclinical testing
lactose
nanoformulations
INTRODUCTION
The use of inhalation therapy for administering drugs finds its origin around 4000 years ago. Datura roots smoke as a bronchodilator was used in India in 2000 BC.1 Vapors of black henbane, which contained anticholinergic compound hyoscyamine were used by physicians in Egypt for treating breathing issues in 1554 BC.2 Since then, many methods were employed as inhalation therapies for administering drugs to treat diseases associated with respiration. However, the development of drug delivery systems to deliver the drugs to the lungs can be traced back to the 1950s when Riker laboratories commercialized a pressurized metereddose inhaler (pMDI) in 1956.3 This paved the way for researchers to introduce drugs for treating asthma and other diseases associated with respiration. With the increasing use of pMDI, the concern related to the depletion of the ozone layer owing to the use of chlorofluorocarbons was postulated in 1974.4 The chlorofluorocarbons were substituted by hydrofluoroalkanes in 1995, which were considered more eco-friendly, and they were assumed not to deplete the ozone layer.5–7 Various drawbacks of pMDIs were responsible for the introduction of dry powder inhaler (DPI) as a drug delivery device.
The drawbacks of pMDIs are:8–11
-
Low solubility
-
Chemical instability
-
Crystal growth phenomenon
-
Instability of suspensions
-
Inaccuracies in metering doses
-
Limitations in drug loading capacities
-
Limited drug targeting capacities
-
Reduced patient compliance owing to the freon effect
The following review discusses in brief the journey of DPIs and their formulation aspect. It also highlights the use of Anderson Cascade impactor.
The first DPI, Spinhaler was introduced in the market by Fison (Ipswich, UK) in 1967.2,12 They are devices that work on the concept of breath actuation, that is, they are activated by the patient’s inspiratory flow rate. These devices offer various advantages over other inhalational drug delivery systems and are known for their effectiveness in the treatment of pulmonary diseases.13 As DPI has several advantages over pMDIs, there has been tremendous growth in the formulation of DPI in the past few decades with several clinical trial phases, and many DPIs are available in the market.14
Pulmonary route of drug administration
The respiratory tract: The respiratory tract is divided into the upper and lower respiratory tract. Nose, nasal cavity, thorax, pharynx, and larynx form the upper respiratory tract, whereas trachea, bronchi, bronchioles, and alveolar region form the lower respiratory tract. The alveolar region is responsible for gaseous exchange. It consists of bronchioles, alveolar ducts, and alveoli.15 Figure 1 describes the human respiratory tract.16
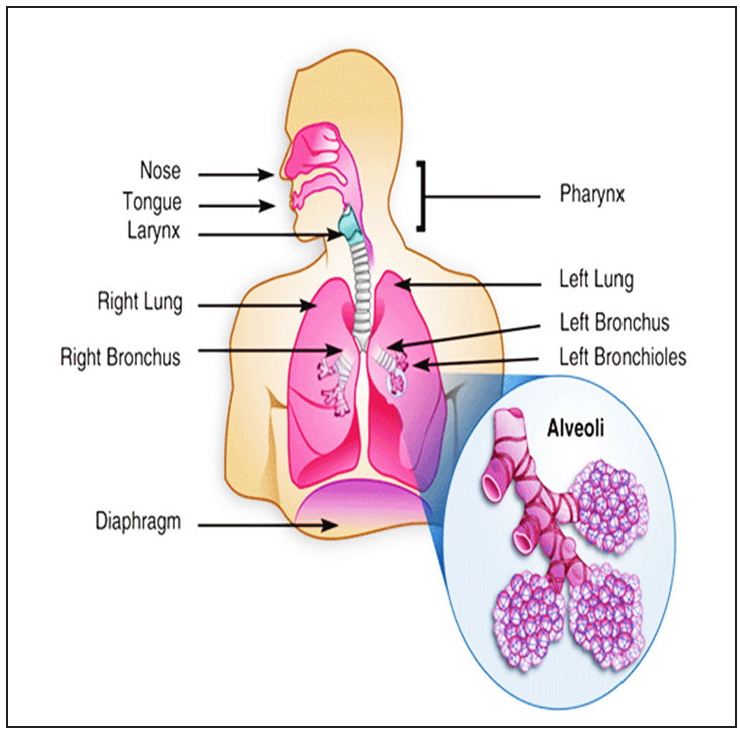
- Human respiratory tract.
The morphometric model of human lungs was proposed by Edward Weibel in 1963 to promote the idea of a quantitative description of pulmonary anatomy and its correlation with physiology. According to this model, the lung is divided into 24 compartments with each compartment analogous to a generation of the model. It speculates that each generation of airways branches symmetrically into two equivalent generations. According to this model generation, 0–16 consist of the trachea-bronchial region and 17–23 comprise the alveolar region.17 Together, these provide a large surface area of approximately 140 m2.18
Delivery of drug to lungs: Over the years, drug delivery to the respiratory tract has gained a lot of attention by researchers and has so far been considered as one of the best alternatives to other routes of drug administration.19 The conventional routes for treating respiratory diseases have various limitations, which have paved the way for researchers to focus on targeting drugs to a specific site. Pulmonary drug delivery offers this advantage of being site-specific, both for systemic and local drug delivery. Many drugs have displayed an appreciable increase in bioavailability post-pulmonary administration. It can be associated with the factors like (1) greater surface area of alveoli, (2) comparatively low metabolic activity, and (3) increased blood flow responsible for the rapid distribution of drugs in the body.20
Delivery of drugs to the lungs offer advantages of both local and systemic drug delivery. Local effects can be observed in treating diseases like asthma and chronic obstructive pulmonary disorder, whereas the natural permeability of the lungs can be beneficial for transferring drugs via systemic routes.21–25
Advantages of a pulmonary drug delivery system:26,27
-
Avoids hepatic first-pass metabolism
-
Noninvasive route of drug administration
-
Rapid onset of action
-
Targeted drug delivery can be achieved
-
Negligible side effects
-
Dose reduction can be accomplished
-
Provides a large surface area for absorption of drugs
While developing formulation for efficient deposition of drugs in the lungs, it is necessary to consider factors like physical, chemical, and physiological properties of inhaled particles, barriers to deposition of drug and absorption of drugs, other barriers such as the thickness of mucus lining, phagocytosis by macrophages, proteolytic degradation, and so on.28,29
Factors governing deposition of particles in the lungs: For a drug to be delivered in the lungs, it must be administered in aerosol form. Drug deposition in the deeper sections of the lungs is governed by various biophysical parameters:
-
Aerodynamic diameter
-
Inspiration pattern of patients
-
Anatomy of the respiratory tract of the patient
Particle size plays a very important role in determining the site of deposition of the drug within the respiratory tract.
Aerodynamic diameter of particle: It is defined as the diameter of a sphere of unit density that has the same terminal settling velocity as the particle under consideration. Similarly, aerosol particles with varying densities and shapes can be categorized according to their aerodynamic properties.
Aerodynamic diameter usually depends on the airflow (particle Reynolds number, Re) and particulate properties, including geometry, size, shape, and density of the particle. With Stokes flow regime of Re <0.1, the aerodynamic diameter of particle (dA) can be calculated as:
dv: Volume equivalent diameter
ρ: Particle density
ρo: Unit density (1g/cm3)
x: Dynamic shape factor
Dynamic shape factor is the ratio of drag force on the particle to the drag force on the particle volume equivalent sphere at the same velocity for the spherical particle; the dynamic shape is 1.30
Deposition of particles in the respiratory tract
Particle deposition occurs when particles come in contact with the respiratory surface and do not ricochet or are not suspended back in the airstream. Particles that remain suspended in the airstream are exhaled out and do not deposit in the respiratory tract. The different mechanisms by which particle deposition occurs and the particle size range of deposition is given in Table 1.
Table 1 gives an overview of various mechanisms of particle deposition and site of deposition of a drug at a specific site, depending on the particle size.
Inertial impaction: It is a velocity-dependent mechanism. Larger particles (>5 μm) follow this principle of drug deposition. It is one of the most common mechanisms of particle deposition. It occurs at bifurcations of the respiratory tract where the change in the direction of airflow is observed, but the particles in the airstream follow the predefined route rather than adjusting to the change in the direction of airflow. This leads to the deposition of particles in the upper respiratory tract (nose, larynx) and conducting airways (trachea, bronchi).29,31
Gravitational Sedimentation: Deposition of particles by sedimentation occurs due to gravitational forces. This process being time-dependent, an increase in the deposition of particles is observed with an increase in the resistance time of that particle in airways and alveoli. The particles which tend to get deposited by this mechanism are usually in the size range of 0.5–3 µm. It is important to consider the fact that particles with diameters < 1 µm have negligible sedimentation velocity, and hence, increasing particle size will eventually increase the settling velocity.23,29
Brownian Diffusion: It is a result of the random motion of particles that are suspended in the aerosol stream and collide with gas molecules. These result in the haphazard motion of particles from aerosol clouds and results in deposition on the walls of the airways. In this mechanism, deposition of particles is inversely proportional to the particle diameter. Hence, it is a very important mechanism for the deposition of particles with a diameter < 0.5 µm deep within the lungs.23,24,29
Dry powder inhaler: These are the devices that store the drug as fine aggregates and deliver the dry powder of medicament in the lungs for its local or systemic effect. The drug is usually stored in a reservoir at the bottom of the inhaler device.32 For delivery of a drug, the patient’s inspiration force plays an important role in initiating the actuation of the inhalation device. However, some DPI devices appear to be relatively independent of the patient’s inspiratory rate.33
DPIs are commonly used for local as well as systemic administration of drugs. Drugs effective in cystic fibrosis, asthma, a chronic obstructive pulmonary disorder, and lung cancer can be administered locally with a noticeable reduction in systemic side effects and localized concentration of drug at the target site.34–39
Delivery devices for dry powders for inhalation: Tables 2, 3, and 4 describe the different types of DPIs.
Table 2 gives an idea of different types of first-generation dry powder inhaler devices along with the mechanism of delivering the drug.
Sr. No | Device | Type | Company | Reservoir system | Mechanism |
---|---|---|---|---|---|
1 | Spinhaler | Single unit dose | Aventis | Capsule | Passive (breath actuated), the drug delivered due to puncturing of the capsule by two pins present in the system. |
2 | HandiHaler | Single unit dose | Boehringer Ingelheim/Pfizer | Capsule | Passive (breath actuated), the drug delivered due to puncturing of the capsule by pins in the system. |
3 | Aerosolizer | Single unit dose | Novartis | Capsule | Passive (breath actuated), the drug delivered due to puncturing of the capsule by four pins in the system. |
4 | Cyclohaler | Single unit dose | Teva | Capsule | Passive (breath actuated), the drug delivered due to puncturing of the capsule by pins in the system. |
5 | Rotahaler | Single unit dose | Glaxo | Capsule | Passive (breath actuated), the drug delivered due to puncturing of the capsule by pins in the system. |
Table 3 gives an overview of different types of second-generation dry powder inhaler devices.
Sr. No. | Device | Type | Company | Reservoir system | Mechanism |
---|---|---|---|---|---|
1 | Turbuhaler | Multiple dose | Astra Zeneca | Powder Reservoir | Passive (breath actuated) |
2 | Clickhaler | Multiple dose | Innovata Biomed | Powder Reservoir | Passive (breath actuated) |
3 | Easyhaler | Multiple dose | Orion Pharma | Powder Reservoir | Passive (breath actuated) |
4 | Novolizer | Multiple dose | Astra Medica (Viatris GmbH) | Cartridge | Passive (breath actuated) |
5 | Pulvinal | Multiple dose | Chiesi | Powder Reservoir | Passive (breath actuated) |
6 | Diskhaler | Multiunit dose | GlaxoSmithKline | Single dose in Blister/Disks | Passive (breath actuated) |
7 | Accuhaler/Diskus | Multiunit dose | GlaxoSmithKline | Single dose in Blister/Disks | Passive (breath actuated) |
8 | Elliptia | Multiunit dose | GlaxoSmithKline | Single dose in Blister/Disks | Passive (breath actuated) |
Table 4 gives an idea of different types of third-generation inhaler devices along with the mechanism of drug delivery.40–48
Sr. No. | Device | Type | Company | Reservoir System | Mechanism |
---|---|---|---|---|---|
1 | Exubera | Multiple dose | Nektar Therapeutics (Pfizer) | - | Active (Compressed Gas) |
2 | Afrezza | Technosphere |
Mankind Corp Inhaler (Dreamboat inhaler) |
Cartridge | Active |
Advantages and disadvantages of dry powder inhalers
Advantages
-
Most DPIs are breath-actuated devices. Hence, the patient’s coordination is not needed.
-
The dosage form is in powdered form which enhances the physicochemical stability of the formulation.
-
The system is environmentally friendly as it does not use propellants for delivering the drug to the lungs.
-
Compact nature makes them easy to carry.
-
Easy to use.
-
Deliver high drug payloads to the lungs.
Disadvantages
-
Manufacturing of DPIs is complex and any errors during preparation might be critical.
-
The emission of dose and deposition efficacy is dependent on the patient’s inspiratory flow.
-
If the inspiration speed is too slow, very little or no dose is emitted from the device.
-
It is easily affected by environmental factors like humidity. Hence, they must be stored in a cool and dry place.
-
Some inhaler devices need to be shaken before use.
-
Expensive as compared to pMDIs.
Ideal properties of dry powder inhaler devices
-
They must be easy to use.
-
They must be economic and compact.
-
Minimum loss of drug must occur during respiration.
-
They must be able to protect against an accidental overdose of the drug.
-
They must provide maximum aerosolization with minimal efforts for asthmatics or small children and geriatrics.
-
They must deliver the drug accurately.
General requirements of dry powder inhaler
-
The particle size of the drug: 1–10 µm.
-
Drug content uniformity.
-
Stability of powder toward different environmental conditions such as humidity, temperature, and so on.52
Formulation strategies for dry powder inhaler
-
Drug carrier: DPIs hold the drug for delivery in either a blister or capsule or a reservoir, where the quantity of the drug is as low as 1 µg and is difficult to deliver. Moreover, the ideal aerodynamic diameter of particles that is desired for the delivery of drugs must range from 1 µm to 5 µm. The problem while using the power of such a small particle size is that it significantly increases cohesiveness and adhesiveness owing to their high surface free energy. This results in poor flow and aerosolization behavior of particles as they are confined to the device when used alone. To improve the flow properties, there is a need to use a carrier whose size range varies from 50μm to 100µm along with the drug. The blending process results in the drug particles adhering to the carrier particles. These carriers act as diluents and eventually improve the flow properties. The problem with this approach is that the increased volume of powder that is inhaled drastically reduces the quantity of drug reaching the lungs.53,54
Process of the detachment of particles from the carrier surface
When a mixture of the drug along with the carrier is inhaled, the process of detachment of drug particles from the carrier surface occurs in three steps:
-
Fluidization of mixture in the airstream
-
A detachment of drug particles or agglomerates from the carrier surface
-
Breakdown of detached agglomerates into primary particles
Once the particles are detached from the carrier surface, they will be deposited in the lungs, whereas the larger particles, that is, carriers, will be swallowed following impact in the mouth and back region of the throat. Figure 2 shows the process of detachment of particles from the carrier surface.55
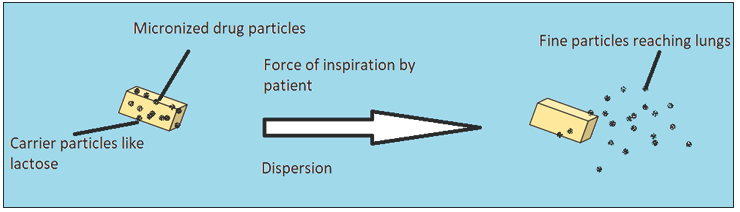
- Detachment of drug particles from the carrier surface.
Carriers used in the formulation of dry powder inhalers:
-
Lactose: It is the most widely used carrier of dry powder for inhalation and is available in various inhalation grades. It has been approved as a carrier in DPIs by the US Food and Drug Administration (FDA). Lactose has been extensively used as a carrier, but its limitations regards to certain drugs and peptides restrict its use as a carrier in such formulations, thus emphasizing the need for alternative carriers for DPIs.56,57
-
Mannitol: This is the best alternative for lactose as a carrier for DPI. It has overcome the problems of using lactose in scenarios where the sugar moiety interacts with the drug (e.g., protein and peptides).58 Another problem observed with lactose is related to patients where, in some cases, lactose intolerance is observed.59 Unlike lactose, mannitol lacks reducing sugar moiety and its less hygroscopicity adds to the advantage when compared to lactose.60 Improved patient compliance owing to its sweet aftertaste is observed.61 Currently, D-mannitol is available as pulmonary diagnostic dry powder inhalation aerosol and therapeutical dry powder inhalation aerosol for the treatment of cystic fibrosis and chronic bronchitis (Bronchitol™) in some countries and has been recently approved by US FDA and Europe, the Middle East, and Africa (EMEA).62
-
Sorbitol: Lactose reacts with lysine residues present in the proteins and generate lactosylated protein molecule.63 Sorbitol is useful in such cases as it serves as a stability enhancer during formulation and does not react with protein, thus forming a stable respirable protein powder. Sorbitol also plays a crucial role in producing respirable powder of Interferon β as stability during jet milling can be achieved due to the presence of Sorbitol.64,65
-
Erythritol: Various fruits and fermented foods have shown the presence of this naturally occurring sugar alcohol. The industrial process of making erythritol includes glucose fermentation.66 Low hygroscopicity, thermal stability, sweet taste, and low toxicity contribute to its efficacy as a carrier for DPIs.67 Its compatibility with a wide range of drugs makes it an interesting candidate for use.68
-
Trehalose: Trehalose dihydrate is a nonreducing sugar. Its use as a carrier in DPIs was reported with drugs like albuterol sulfate, ipratropium bromide, monohydrate disodium cromogylate, and fluticasone.62
Integration of Formulation into a device
On blending the drug with the carrier, it is filled into a reservoir system which is then placed in the inhaler device. Various systems are:
-
Capsules: These are used by the first-generation DPIs, where the powder is filled in a capsule and the capsule is loaded into the device before use. Drug delivery is achieved either by the opening of the capsule within the device or by piercing the walls of the capsule with the help of pins present in the device.47 The material used for making capsules is hydroxypropyl methylcellulose and hard gelatine. The use of hydroxypropyl methylcellulose has gained attention recently as it contains less water as compared to gelatin and is useful in the case of hygroscopic drugs69 Also, the fragmentation due to the piercing of pins is less, even at low humidity.70 Although the capsule-based system is comparatively inconvenient for single-dose containers, certain modifications like using capsule size larger than three and using mechanisms such as the opening of capsule rather than piercing may prove to be beneficial and also serve as an area for further innovations.71
-
Blister system: This reservoir system is used by DPI devices like Diskhaler. In such a system, on actuation, the upper and lower surfaces of blisters where the drug is placed are pierced by a needle and the drug is dispersed into the airstream as the patient inhales. The disk then rotates to introduce the next blister for piercing on repriming.47
-
Carrier-free particles: Formulation of carrier-free particles overcomes the major disadvantages of carrier-based systems, such as blend uniformity issues, and it is also beneficial in cases where delivery of high payload of the drug is essential, especially in the case of antibiotics. In this strategy, the drug is available either as a single compound or multi-compound composite of encapsulated particles. The particle size plays a very important role in formulating carrier-free systems. The particle size must be below 5 µm. With the view on improving the efficacy, different techniques have been used to formulate respirable drug particles. These include milling, crystallization, spray drying, spray freeze drying, supercritical fluid technology, and antisolvent technology. Crystallization and milling are not suitable for processing drugs for pulmonary use as they cannot produce particles with optimum shape, size, and particles with low surface energy.26,52,72
Animal models used to access the pulmonary delivery of aerosol during preclinical phase
Different animals have been used to determine the effect of inhaled material. The different parameters that are considered while selecting animals are cost, disease pathology, and comparison of the immunological response to humans.18,73
Although the data obtained from preclinical studies are valuable, its direct correlation to effects in humans is difficult since animals have different nasal, tracheobronchial, and deep lung regions than humans, and these vary in different species too. It is, therefore expected that the anatomy of the respiratory tract of animal models should draw a significant correlation to the human respiratory tract. The breathing pattern of humans is different from rodents. Rodents cannot breathe through mouth, whereas adult humans can breathe through mouth as well as nose. As rodents are obligate nose breathers, the data obtained for lung deposition has limited relevance with data for humans. The data for animals are obtained by nose-only exposure system and whole-body exposure system. The absence of respiratory bronchioles in some animals shows limitations while comparing data of drug deposition in different areas of lungs when compared with humans.74 The cell lining varies in animal models too, for example, human alveolar macrophages are estimated to be more in number and are also larger as compared to rodents.75
Preclinical inhalation methodologies
The lack of preclinical pulmonary devices is one of the major hindrances in evaluating the efficacy of DPI devices. The devices used for human beings work differently than those used in preclinical models. There are two different categories of devices that are used for preclinical studies viz. passive inhalation devices and direct inhalation devices. In passive inhalation devices, the animal’s respiratory force is considered for delivering content, whereas for direct inhalation devices the aerosol is forced through the upper respiratory tract, which is usually under anesthetic condition.
Passive inhalation devices utilize whole-body inhalation chamber and head and nose only chambers. For animals such as rabbits and rodents, the drug passes through nasal cavities before reaching the lungs.76 They being obligate nose breathers with different nasal anatomy, the amount of drug deposition in the lungs will vary and will also be different from humans. In the whole-body chamber, the animal is exposed to aerosol. The benefit of such a system is that they can house many animals at once and access to food and water can be given. Examples of such devices include Glas-col®, inhalation exposure system, and other units from TSE systems and Shibaba Biotechnology.77,78 Despite various advantages offered by the whole-body exposure system, it has certain flaws like extra pulmonary exposure via other routes like skin and oral/GI tract. It also requires a large dose and shows the difficulty in quantification and characterization of the amount of drug delivered in the lungs. Such chambers can be used for studying environmental exposure that needs long-term dosing. Figure 379 gives an idea of the whole-body exposure system.
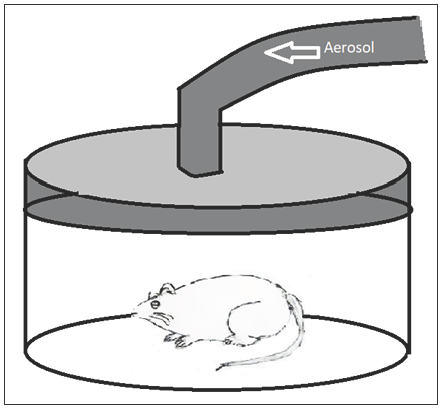
- Whole body exposure chamber.
In head and nose-only exposure chambers, the animals are held in such a way that they are immobilized and only animals’ nose or head is exposed to the drug present in the airstream. Figure 4 gives an idea of the head and nose-only exposure system.79
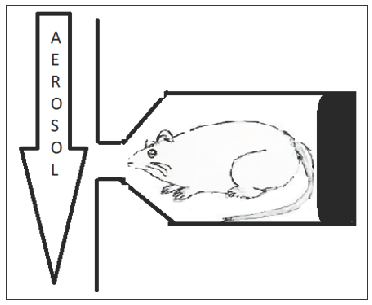
- Head and nose-only exposure chamber.
The benefit of such a system is that it reduces extra pulmonary exposure of the drug to other routes, thus minimizing the amount of API and excipients required for the experiment. The problems with this system are the deposition of almost 80%–90% of the drug in the nasopharynx region and upper respiratory tract.80,81 Quantification of the dose is difficult as less quantity of drug reaches the lungs; moreover, this system induces stress on animals owing to mobility issues and deprivation of food and water access.
Direct inhalation devices include intrathecal methods for the delivery of drugs. In such systems, the animals are anesthetized and aerosol is forced into the trachea. In these systems, quantification of drug delivered in the lungs is easier.76 Tools for delivering dry powders include the Penn Century Insufflators™ and Biolite intubation system (Braintree Scientific Inc).82,83 Figure 5 shows an intubated rodent and Penn Century Insufflator device.79
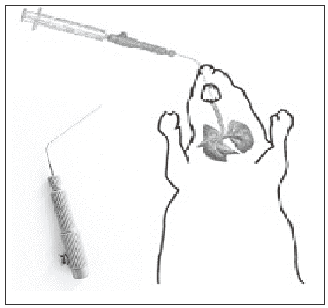
- Intubated rodent and Penn Century Insufflator device.
The anesthetized rodent is intubated with the insufflator tube or catheter up to the first bifurcation of the lungs. Dry powder is then aerosolized into the lungs with the help of a syringe or pipette bulb. With this system, repeated dosing and long-term studies can be carried out.83
Andersen cascade impactor
The use of Andersen Cascade Impactor to assess the size distribution of particles has been reported extensively. To determine the particle size, usually the formulation in the form of the aerosol is aspirated through a series of impaction stages in order of their decreasing pore size.
Development of Andersen Impactor:
Andersen Impactor is a widely used cascade impactor for determining the particle size distribution. The original design was framed by Andersen. The design consisted of various stages arranged in series with increasing efficacy. Each series consisted of several identical circular accelerating jets (usually 400) arranged in circular patterns facing toward an 82 mm diameter collection plate. When the aerosol impinges the plate, it moves radially outward to the edge of the plate and moves to the next plate taking a turn of 180°. There are different versions of this instrument. The different developmental stages of this sampler are:84–86
-
A six-stage cascade impactor was developed by Andersen in 1958 to collect airborne bacteria. In this system, the bacteria impacted on the surface of the agar in a petri dish which was calibrated by counting and sizing of collected wax spheres by optical microscopy. The same design was applied in 1966 to collect nonviable particles on stainless steel or glass impaction plate.
-
The sampler was redesigned in 1970 to further categorize incoming dust into eight different fractions (Stages 0–7) with the inclusion of an integrated backup filter. This version was named MK I.
-
Later in 1977, the MK II version of the sampler was introduced with contributions from McFarland et al.86 (1977) in 1977 who emphasized the need to redesign the top two stages and apply a pre-impactor.
Various studies on impactors have concluded that impactors can be designed according to the size specificity criterion.
Operational principle of cascade impactor
The functioning of the cascade impactor is based on the principle of inertial impaction. Each stage of the impactor consists of a single or series of nozzles or jet, through which the sample is introduced in the form of aerosol and directed toward the collection plate of that stage. The chances of particles impacting the stage depend on its aerodynamic particle size. Smaller particles will pass to the next stage owing to less inertia. The process is repeated for the next stages, too. The different stages are arranged in decreasing order of particle size. With the reduction of the orifice of the jet, the increase in velocity of air is observed which enables the collection of finer particles. If any particle remains, it is collected after the filter. Figure 6 gives a brief view of the different stages of Anderson cascade impactor and possible sites of drug deposition.87
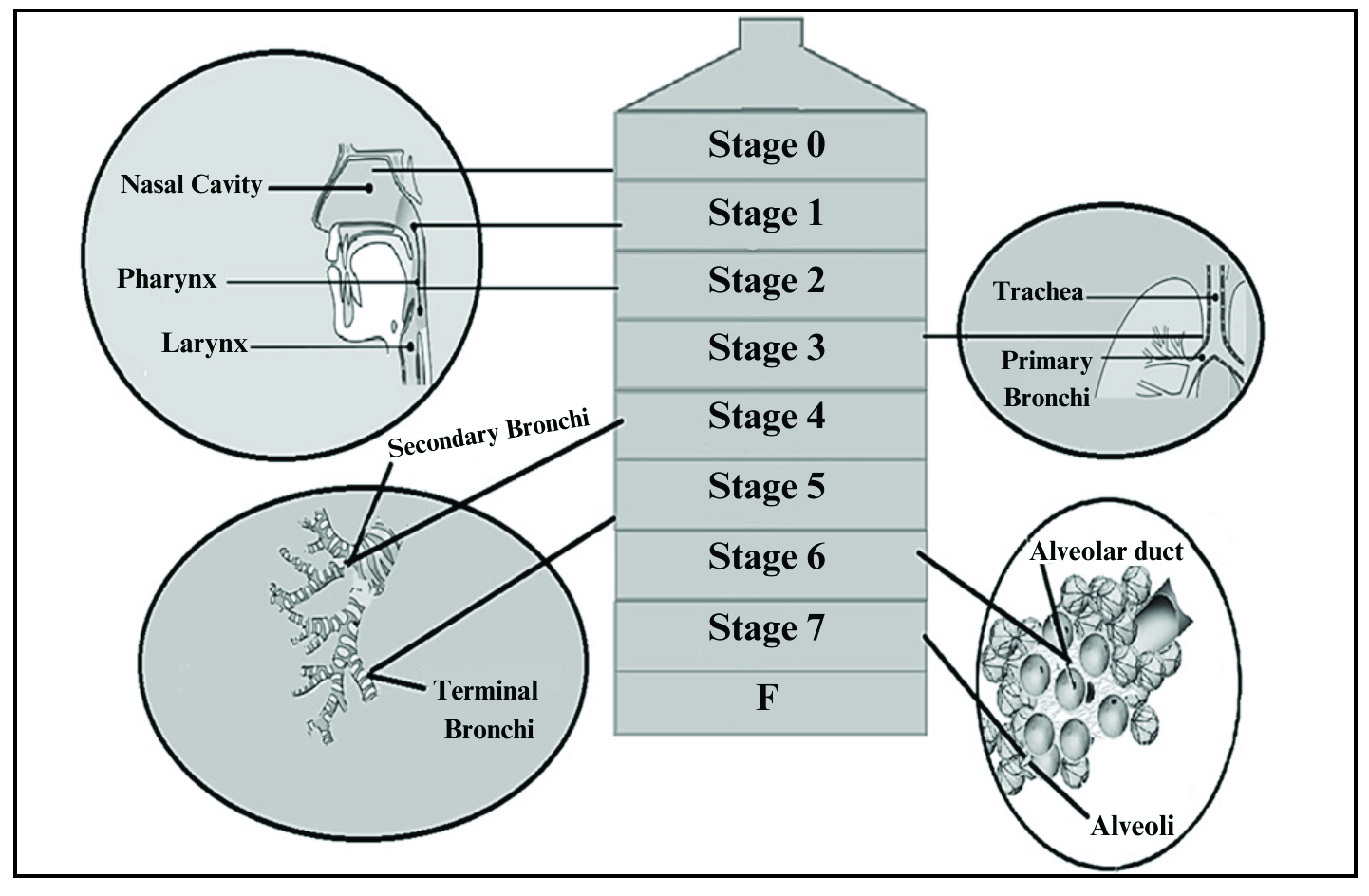
- Relation between Andersen Cascade Impactor and respiratory tract.
Table 5 gives an idea of the relation between Andersen Cascade impactor and possible particle size deposition within the respiratory tract.
Sr No | Stages of anderson cascade impactor | Particle size per stage in µm | Probable site of drug deposition | Aerosol size in µm |
---|---|---|---|---|
1 | Stage 0 | 9.0–14.0 | Upper respiratory tract | 9.0–10.0 |
2 | Stage 1 | 5.8–9.0 | Upper respiratory tract | 5.8–8.9 |
3 | Stage 2 | 4.7–5.8 | Pharynx and larynx | 4.7–5.7 |
4 | Stage 3 | 3.3–4.7 | Trachea and primary bronchi | 3.3–4.6 |
5 | Stage 4 | 2.1–3.3 | Secondary bronchi | 2.1–3.2 |
6 | Stage 5 | 1.1–2.1 | Terminal bronchi | 1.1–2.0 |
7 | Stage 6 | 0.65–1.1 | Alveoli | 0.7–1 |
8 | Stage 7 | 0.43–0.65 | Alveoli | 0.4–0.6 |
Filter | Below 0.4 |
CONCLUSION
DPIs have been an interesting area of research with newer trends like nanoparticulate systems, self-aggregating systems, microparticulate systems, liposomal drug delivery, and delivery of proteins and peptides gaining attention in recent years. The future of DPIs looks promising with researchers experimenting extensively on the use of different formulation strategies as well as the use of different excipients for safe and efficacious delivery of drugs directly to the lung in the form of dry powders for inhalation.
Authors’ contributions
HSM has contributed to concept of the review article and has revised it critically for important intellectual content; PSP has collected the data and drafted the article.
Ethical approval
Institutional Review Board approval is not required.
Declaration of patient consent
Patient's consent not required as there are no patients in this study.
Financial support and sponsorship
Nil.
Conflicts of interest
There are no conflicts of interest.
Use of artificial intelligence (AI)-assisted technology for manuscript preparation
The authors confirm that there was no use of artificial intelligence (AI)-assisted technology for assisting in the writing or editing of the manuscript and no images were manipulated using AI.
REFERENCES
- History of aerosol therapy: Liquid nebulization to MDIs to DPIs. Respir Care. 2005;50:1139-50.
- [PubMed] [Google Scholar]
- Inhalation therapy: An historical review. Prim Care Respir J. 2007;16:71-81.
- [CrossRef] [PubMed] [Google Scholar]
- A brief history of inhaled asthma therapy over the last fifty years. Prim Care Respir J. 2006;15:326-31.
- [CrossRef] [PubMed] [Google Scholar]
- Stratospheric sink for chlorofluoromethanes: Chlorine atom-catalyzed destruction of ozone. Nature. 1974;249:810-2.
- [Google Scholar]
- UNEP. The Montreal Protocol on substances that deplete the ozone layer. Available from: http://ozone.unep.org/pdfs/Montreal-Protocol2000.pdf [Last accessed 26 Mar 2020].
- Use of ozone-depleting substances; removal of essential-use designations. Final rule. Fed Regist. 2005;70:17167-92.
- [Google Scholar]
- Use of ozone-depleting substances; removal of essential-use designation (flunisolide, etc.) Final rule. Fed Regist. 2010;75:19213-41.
- [Google Scholar]
- The influence of formulation variables on the performance of alternative propellant-driven metered dose inhalers. Adv Drug Deliv Rev. 2003;55:807-28.
- [CrossRef] [PubMed] [Google Scholar]
- Chemical stability in pressurized inhalers formulated as solutions. J Biopharm Sci. 1992;3:41-7.
- [Google Scholar]
- Surfactant promoted crystal growth of micronized methylprednisolone in trichloromonofluoromethane. Int J Pharm. 1994;110:9-19.
- [CrossRef] [Google Scholar]
- Interfacial phenomena and phase behaviour in metered-dose inhaler formulations. In: Hickey AJ, ed. Inhalation aerosols (94). New York: Marcel Dekker; 1996. p. :385-415.
- [Google Scholar]
- Dry powder aerosols. I. A new powder inhalation device. J Pharm Sci. 1971;60:1559-64.
- [CrossRef] [PubMed] [Google Scholar]
- The inhalation device influences lung deposition and bronchodilating effect of terbutaline. Am J Respir Crit Care Med. 1996;153:1636-40.
- [CrossRef] [PubMed] [Google Scholar]
- The History of Therapeutic Aerosols: A Chronological Review. J Aerosol Med Pulm Drug Deliv. 2017;30:20-41.
- [CrossRef] [PubMed] [PubMed Central] [Google Scholar]
- Pulmonary drug delivery. In: Hillery AM, Llyod AW, Swarbick J, eds. Drug delivery and targeting for pharmacist and pharmaceutical scientist. London: Taylor and Francis; 2001. p. :244-73.
- [Google Scholar]
- Wikimedia Commons. Available from: https://commons.wikimedia.org/wiki/File:Human_Lungs.gif [Last accessed 29 Mar 2020]
- Geometric and dimensional airway models of conductive, transipiratory and respiratory zones of the human lungs. In: Weibel ER, ed. Morphometry of Human Lungs. Vol volume 1. Berlin: Springer, Berlin Heidelberg; 1963. p. :136-42.
- [Google Scholar]
- Preclinical models for pulmonary drug delivery. Expert Opin Drug Deliv. 2009;6:1231-45.
- [CrossRef] [PubMed] [Google Scholar]
- Treating systemic diseases via the lung. J Aerosol Med. 2001;14 Suppl 1:S51-8.
- [CrossRef] [PubMed] [Google Scholar]
- Pulmonary absorption of peptides and proteins. Drug Delivery. 1995;2:1-20.
- [CrossRef] [Google Scholar]
- Pharmacokinetics and relative bioavailability of salbutamol metered-dose inhaler in healthy volunteers. Acta Pharmacol Sin. 2002;23:663-6.
- [PubMed] [Google Scholar]
- The lungs as a portal of entry for systemic drug delivery. Proc Am Thorac Soc. 2004;1:338-44.
- [CrossRef] [PubMed] [Google Scholar]
- Pulmonary drug delivery. In: Aulton ME, Taylor KMG, eds. Aulton’s pharmaceutics: The design and manufacture of medicines. London: Elsevier Health Sciences; 2013. p. :638-56.
- [Google Scholar]
- particulate interactions in dry powder formulation for inhalation (1st edition). London: CRC Press, Taylor & Francis; 2000.
- The expanding role of aerosols in systemic drug delivery, gene therapy, and vaccination. Respir Care. 2005;50:1161-76.
- [PubMed] [Google Scholar]
- Pulmonary drug delivery strategies: A concise, systematic review. Lung India. 2012;29:44-9.
- [CrossRef] [PubMed] [PubMed Central] [Google Scholar]
- Recent advances in pulmonary drug delivery system: A review. Int J of App Pharm. 2010;2:27-31.
- [Google Scholar]
- The clinical relevance of dry powder inhaler performance for drug delivery. Respir Med. 2014;108:1195-203.
- [CrossRef] [PubMed] [Google Scholar]
- Clinical perspectives on pulmonary systemic and macromolecular delivery. Adv Drug Deliv Rev. 2006;58:996-1008.
- [CrossRef] [PubMed] [Google Scholar]
- Particle engineering for pulmonary drug delivery. Pharm Res. 2007;24:411-37.
- [CrossRef] [PubMed] [Google Scholar]
- A monte carlo calculation of the deposition efficiency of inhaled particles in lower airways. J Aerosol Sci. 1994;25:711-21.
- [CrossRef] [Google Scholar]
- Counseling about chlorofluorocarbon-free inhalers. Am J Health Syst Pharm. 1998;55:226-7.
- [CrossRef] [PubMed] [Google Scholar]
- Lung deposition from the Turbuhaler in children with cystic fibrosis. Eur Respir J. 1997;10:2023-8.
- [CrossRef] [PubMed] [Google Scholar]
- Nebulized gentamicin in children and adolescents with cystic fibrosis. Aust Paediatr J. 1984;20:43-5.
- [CrossRef] [PubMed] [Google Scholar]
- The 1997 Asthma Management Guidelines and therapeutic issues relating to the treatment of asthma. National Heart, Lung, and Blood Institute. Chest. 1999;115:210-7.
- [CrossRef] [Google Scholar]
- Inhaled antibiotics for long-term therapy in cystic fibrosis. Cochrane Database Syst Rev 2011:CD001021.
- [CrossRef] [Google Scholar]
- Aerosol delivery of liposomal all-trans-retinoic acid to the lungs. Cancer Chemother Pharmacol. 1999;43:277-83.
- [CrossRef] [PubMed] [Google Scholar]
- Targeting delivery of aerosols to different lung regions. J Aerosol Med. 2002;15:179-88.
- [CrossRef] [PubMed] [Google Scholar]
- Development of inhalational agents for oncologic use. J Clin Oncol. 2001;19:1839-47.
- [CrossRef] [PubMed] [Google Scholar]
- Advancements in dry powder delivery to the lung. Drug Dev Ind Pharm. 2008;34:948-59.
- [CrossRef] [PubMed] [Google Scholar]
- Developing an efficient and reliable dry powder inhaler for pulmonary drug delivery--a review for multidisciplinary researchers. Med Eng Phys. 2012;34:409-27.
- [CrossRef] [PubMed] [Google Scholar]
- The inhalers of the future? A review of dry powder devices on the market today. Pulm Pharmacol Ther. 2003;16:79-95.
- [CrossRef] [PubMed] [Google Scholar]
- Dry powder inhalers (DPIs)--a review of device reliability and innovation. Int J Pharm. 2008;360:1-11.
- [CrossRef] [PubMed] [Google Scholar]
- The ideal inhaler: Design and characteristics to improve outcomes. Respir Med. 2004;98 Suppl A:S10-6.
- [CrossRef] [PubMed] [Google Scholar]
- Effect of design on the performance of a dry powder inhaler using computational fluid dynamics. Part 1: Grid structure and mouthpiece length. J Pharm Sci. 2004;93:2863-76.
- [CrossRef] [Google Scholar]
- The Diskus: A review of its position among dry powder inhaler devices. Int J Clin Pract. 2007;61:1022-36.
- [CrossRef] [PubMed] [PubMed Central] [Google Scholar]
- Closer to an 'ideal inhaler' with the Easyhaler: An innovative dry powder inhaler. Clin Drug Investig. 2006;26:175-83.
- [CrossRef] [PubMed] [Google Scholar]
- Not all asthma inhalers are the same: Factors to consider when prescribing an inhaler. Prim Care Respir J. 2009;18:243-9.
- [CrossRef] [PubMed] [Google Scholar]
- Latest advances in the development of dry powder inhalers. Pharm Sci Technol Today. 2000;3:246-256.
- [CrossRef] [PubMed] [Google Scholar]
- Dry powder inhaler: An advance technique for pulmonary drug delivery system. IJPCS. 2012;1:1027-34.
- [Google Scholar]
- Deposition of inhaled particles in human respiratory tract in the size range 0.005–15µm. J Aerosol Sci. 2012;17:811-25.
- [Google Scholar]
- Drug delivery to the lungs from dry powder inhalers. Curr Opin Pulm Med. 2003;9(Suppl 1):S17-20.
- [CrossRef] [PubMed] [Google Scholar]
- Recent advances in the fundamental understanding of adhesive mixtures for inhalation. Curr Pharm Des. 2015;21:5900-14.
- [CrossRef] [PubMed] [Google Scholar]
- Alternative carriers in dry powder inhaler formulations. Drug Discov Today. 2014;19:618-26.
- [CrossRef] [PubMed] [Google Scholar]
- Alternative sugars as potential carriers for dry powder inhalations. Int J Pharm. 2004;270:297-306.
- [CrossRef] [PubMed] [Google Scholar]
- Imagine the Superiority of Dry Powder Inhalers from Carrier Engineering. J Drug Deliv. 2018;2018:5635010.
- [CrossRef] [PubMed] [PubMed Central] [Google Scholar]
- Hypersensitivity reaction after inhalation of a lactose-containing dry powder inhaler. J Pediatr Pharmacol Ther. 2014;19:206-11.
- [CrossRef] [PubMed] [PubMed Central] [Google Scholar]
- Influence of carrier on the performance of dry powder inhalers. Int J Pharm. 2007;334:85-91.
- [CrossRef] [PubMed] [Google Scholar]
- The enhanced aerosol performance of salbutamol from dry powders containing engineered mannitol as excipient. Int J Pharm. 2010;392:178-88.
- [CrossRef] [PubMed] [Google Scholar]
- Dry powder aerosols generated by standardized entrainment tubes from alternative sugar blends: 3. Trehalose dihydrate and D-mannitol carriers. J Pharm Sci. 2010;99:3430-41.
- [CrossRef] [PubMed] [Google Scholar]
- Carrier-based strategies for targeting protein and peptide drugs to the lungs. AAPS J. 2005;7:E20-41.
- [CrossRef] [PubMed] [PubMed Central] [Google Scholar]
- Pharmaceutical aerosol formulation of solid polypeptide microparticles and method for the preparation thereof. World Patent. 1991;9,116,038
- [Google Scholar]
- Erythritol: Crystal growth from the melt. Int J Pharm. 2010;388:129-35.
- [CrossRef] [PubMed] [Google Scholar]
- Erythritol: An interpretive summary of biochemical, metabolic, toxicological and clinical data. Food Chem Toxicol. 1998;36:1139-74.
- [CrossRef] [PubMed] [Google Scholar]
- Development of directly compressible powders via co-spray drying. Eur J Pharm Biopharm. 2007;67:220-6.
- [CrossRef] [PubMed] [Google Scholar]
- Recent advances in capsule-based dry powder inhaler technology. Multidiscip Respir Med. 2017;12:11.
- [CrossRef] [PubMed] [PubMed Central] [Google Scholar]
- A comparison of the puncturing properties of gelatin and hypromellose capsules for use in dry powder inhalers. Drug Dev Ind Pharm. 2008;34:870-6.
- [CrossRef] [PubMed] [Google Scholar]
- Capsule dry powder inhalers – time to innovate?. 2016. Available from: https://www.team-consulting.com/insights/capsule-dry-powder-inhalers-time-to-innovate [Last accessed 16 May 2020].
- Dry powders for oral inhalation free of lactose carrier particles. Adv Drug Deliv Rev. 2014;75:32-52.
- [CrossRef] [PubMed] [Google Scholar]
- The relevance of animal models for aerosol studies. J Aerosol Med Pulm Drug Deliv. 2008;21:113-24.
- [CrossRef] [PubMed] [Google Scholar]
- Dosimetry considerations for animal aerosol inhalation studies. Biomarkers. 2009;14 Suppl 1:63-6.
- [CrossRef] [PubMed] [Google Scholar]
- Allometric relationships of cell numbers and size in the mammalian lung. Am J Respir Cell Mol Biol. 1992;6:235-43.
- [CrossRef] [PubMed] [Google Scholar]
- In vitro evaluation of the DP-4M PennCentury insufflator. Eur J Pharm Biopharm. 2014;88:153-9.
- [CrossRef] [PubMed] [Google Scholar]
- Whole-body nanoparticle aerosol inhalation exposures. J Vis Exp 2013:e50263.
- [CrossRef] [Google Scholar]
- A Study on Subchronic Inhalation Toxicity of 1-Chloropropane. Toxicol Res. 2015;31:393-402.
- [CrossRef] [PubMed] [PubMed Central] [Google Scholar]
- Challenges associated with pulmonary delivery of therapeutic dry powders for preclinical testing. KONA Powder Part J 2018:1-16.
- [Google Scholar]
- A hand-held apparatus for "nose-only" exposure of mice to inhalable microparticles as a dry powder inhalation targeting lung and airway macrophages. Eur J Pharm Sci. 2008;34:56-65.
- [CrossRef] [PubMed] [Google Scholar]
- Regional particle size dependent deposition of inhaled aerosols in rats and mice. Inhal Toxicol. 2012;24:27-35.
- [CrossRef] [PubMed] [Google Scholar]
- Improved method of in vivo respiratory-gated micro-CT imaging. Phys Med Biol. 2004;49:4163-72.
- [CrossRef] [PubMed] [Google Scholar]
- Dry-powder pulmonary insufflation in the mouse for application to vaccine or drug studies. Tuberculosis (Edinb). 2009;89:371-7.
- [CrossRef] [PubMed] [Google Scholar]
- New sampler for the collection, sizing, and enumeration of viable airborne particles. J Bacteriol. 1958;76:471-84.
- [CrossRef] [PubMed] [Google Scholar]
- The Andersen Impactor: Calibration, Wall losses and Numerical Simulations. J Aerosol Sci. 1989;20:67-90.
- [Google Scholar]
- Wind tunnel evaluation of a modified Andersen impactor and all-weather sampler inlet. Atmos Enviv. 1977;11:535-9.
- [Google Scholar]
- Formulation and product development of pressurised metered dose inhaler: An overview. Pharmatutor. 2015;3:53-64.
- [Google Scholar]