Translate this page into:
Effect of Sleep Restriction during Pregnancy on Fetal Brain Programming and Neurocognitive Development of Offspring: A Review
Address for correspondence Kamalesh K Gulia, PhD, Scientist G, Incharge Division of Sleep Research, Department of Applied Biology, Biomedical Technology Wing, Satelmond Palace, Sree Chitra Tirunal Institute for Medical Sciences and Technology, Trivandrum-695012, Kerala, India (e-mail: kkguliak@hotmail.com).
This article was originally published by Thieme Medical and Scientific Publishers Pvt. Ltd. and was migrated to Scientific Scholar after the change of Publisher.
Abstract
We spend one-third of our lives in sleep, yet the core function of it still remains an enigma due to underlying complex neural processing in this altered state of consciousness. Sleep requirement varies with phase of development. Neonates spent about 85% of their time in sleep, which is polyphasic in nature. Gradually, this pattern takes the shape of a monophasic sleep in adolescents and adults, with changing micro- and macroarchitecture in every phase. Deprivation of sleep in adults impairs learning and memory, and reduces theta coherence among hippocampus and amygdale during sleep. However, sleep loss during pregnancy can affect the ontogenetic development of networks for sleep–wakefulness and the cognitive development of offspring. Even in normal pregnancy, poor sleep quality, reduced rapid eye movement (REM) sleep, and sleep fragmentation are common observation during the last trimester of pregnancy. Delta power, a marker for the homeostatic drive for sleep, in the NREM sleep during the last trimester of pregnancy and postpartum is increased. However, further sleep loss during late pregnancy is a growing concern. Neonates that are born to the total sleep-restricted dams present significant alterations in their emotional development (symptoms of hyperactivity, increased risk-taking behavior during periadolescence) and immature sleep–wakefulness patterns. The REM sleep restriction during late pregnancy elicits depressionlike traits in neonates, which persist until middle age. For a healthy development of brain and body, thorough understanding of the dynamic nature of sleep in relation to age and state (pregnancy) is instrumental in preventing the above-mentioned conditions of prenatal origin. Although sleep is essential for an active brain (for work during day), it remains an underestimated phenomenon. This review highlights the importance of sleep during pregnancy for a healthy brain network programming in offspring.
Keywords
REM sleep restriction
total sleep restriction
hyperactivity
depression
sleep loss during pregnancy
cognition
human
rat
behavior
Introduction
Sleep is an integral component of our life, yet it remains an enigma due to underlying complex neural processing in this altered state of consciousness. After the discovery of the rapid eye movement (REM) sleep in 1953, it was categorized into two distinct states, nonrapid eye movement (NREM) and REM sleep.1 Subsequently, there was tremendous progress in understanding neurophysiological correlates of the NREM and REM sleep.2,3 It was soon realized that sleep loss is a common problem in the current 24 × 7 societies, due to changing lifestyles. Further evidences from human and animal studies indicate that sleep loss is a potential risk of hypertension, metabolic disorders, insulin resistance, cardiovascular diseases, neurocognitive decline, and even cancer. Almost every function of the body gets affected by lack of sleep. It is emphasized that sleep is a dynamic process that undergoes quantitative and qualitative changes from birth until the fag end of life.
In the 21st century, concerns were raised on poor sleep quality during pregnancy and adverse pregnancy outcomes.4,5,6 Even though several factors including malnutrition, substance abuse (cocaine, marijuana, etc.), alcohol consumption, oral hygiene, human immunodeficiency virus (HIV)/acquired immunodeficiency virus (AIDS), infection, stress, and smoking during pregnancy were identified risk factors for the development of fetus, sleep loss during pregnancy as a risk factor was never given importance (►Fig. 1). World Health Organization has reported that about 10% of women during pregnancy and 13% of women who have just given birth to babies experience mental disorders including anxiety and depression (www.who.int/mental_health/maternal-children).7 In developing nations, this percentage escalates by another 5 to 6%. Globally 10 to 20% of children and adolescents also experience mental disorders. These neuropsychiatric conditions are the leading cause of disabilities in youngsters and they severely influence their development, educational attainments, and abilities to live fulfilling and productive lives (www.who.int/mental_health/maternal-children).7 It is always a difficult task to conduct controlled sleep deprivation experimental studies during pregnancy in human subjects due to the ethical and practical reasons. Amidst this scenario, an extensive study was initiated in the rodent model to study the effects of sleep deprivation during pregnancy on the cognitive development of offspring under the Cognitive Science Research Initiative program of the Department of Science and Technology (India) in 2012.8,9,10,11,12,13,14,15,16,17,18
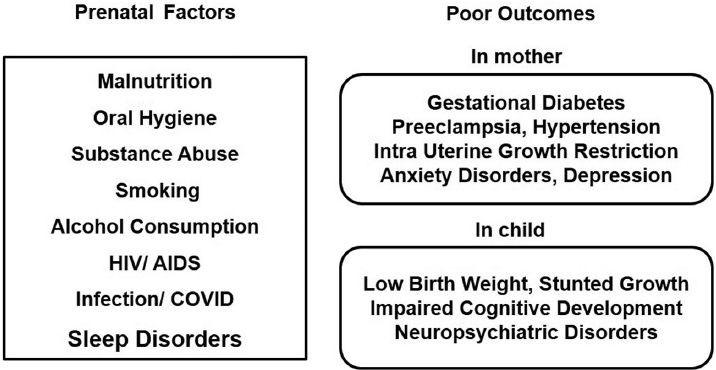
- Factors during pregnancy for poor outcomes on maternal and child health.
Rat Model to Study Effects of Sleep Restriction during Pregnancy
Even though rats are nocturnal animals, they are accepted models for studies involving sleep restriction during pregnancy and ontogenetic neurocognitive development in offsprings.8,9,10,11,12,13,14,15,16,17,18 Similar to humans, pregnancy in rats consists of three trimesters with a total gestation period of 3 weeks. The developmental profile of sleep–wakefulness (S-W) in normal rat dams was assessed by recording electroencephalogram (EEG) and electromyogram (EMG) for 24 hours, starting from prepregnancy days and during pregnancy to postpartum (lactation) and postweaning (PW) days, along with monitoring of their anxiety.11 For this, female rats were chronically implanted with EEG and EMG electrodes under anesthesia and were allowed to recover completely.11 After complete postoperative recovery, 24-hour control recordings of their S-W were taken on 2 consecutive days. These female rats were subjected to mating with age-matched males. This is followed by recording their S-W on various gestational days of pregnancy (GD), postpartum days (PD), and PW were taken, some of which are shown in this article. Sleep fragmentation was observed during the third trimester of pregnancy (GD 19) and postpartum days (PD 4, PD 10) with a concomitant rise in the NREM sleep delta power (►Fig. 2). Delta power during the NREM sleep is a measure of the homeostatic drive for sleep. Delta power in the NREM sleep was calculated from EEG traces, using a fast Fourier transform. Increased NREM sleep delta power during late pregnancy–lactation continuum was evident as a result of sleep fragmentation (►Fig. 2). During normal pregnancy, the postpartum sleep and anxiety decreased compared to the antepartum levels.11

- Pattern of nonrapid eye movement (NREM) sleep and delta power over day and night during pregnancy and after parturition in rats. (A) NREM sleep (%) in 3 hourly bins across day and night for control (C), gestational day 19 (GD19), postdelivery day 4 (PD4), 10 (PD10), and postweaning day 7 (PW7). In the horizontal axis, the dark bar denotes dark period beginning at 6 p.m. and the light bar shows light period beginning at 6 a.m. (B) Normalized delta power is shown for respective bins. (Adapted from Sivadas et al.11)
In the rat model, during the last trimester of pregnancy (GD 15–20), REM sleep restriction (REMSR) was carried out using the classical platform method along with a sham control for 22 h/d from 11 a.m. to 9 a.m. subsequent day (8), whereas the total sleep restriction (TSR) was carried out by the gentle handling procedure wherein the rats were manually woken up whenever they got drowsy (9 a.m. to 2 p.m.).9,10 The effects of REMSR and TSR were studied in the rat model for (1) vocalization pattern to assess the emotional development in pups from postnatal days (PNDs) 1 to 21,8,9,12 (2) ontogenetic profile of S-W from PNDs 1 to 21 and mathematical modelling for developmental assessment,13,14,15 and (3) behavior testing of phenotypic traits of anxiety, depression, etc., for alteration in cognitive development during periadolescence (PNDs 26–46) in three groups.9,10
Cries as Initial Markers of Emotional Development in Human Neonates
Cry (vocalization) at the time of birth in human is the first robust acoustic signal that marks successful switching from the fetal life in amniotic fluid to an air-breathing life.19 The Apgar score in neonates consisting of five features (appearance, pulse, grimace, activity, and respiration) provides a quick assessment of their health profile at the time of birth. Since the language is not developed in newborn, cry remains an important signal for caregivers/mothers and play important biological functions initiating mother–neonate interactions and care giving, which is crucial for optimal physiological and psychological development of infants.
Calls in human babies are marked by phonation, hypophonation, and hyperphonation. An altered vocalizing pattern with abnormal calls is related to brain damage in human babies.20 Hyperphonation, which is marked by the shifts in pitch, is relevant for translational analysis of neurobehavioral dysregulation assessment in human infants. The two-component step calls of rat pups appear analogous to hyperphonation of human babies. Human studies have linked maternal stress with adverse neural developmental in children.21 The decreased REM sleep in pregnant women increased the risk of postpartum depression in mothers.5
Cries as robust acoustic signals contain hidden information about their state of well-being.19,20,22,23 For new parents and caregivers, it is a huge challenge to decode the underlying message in cries. It is a well-known fact that language is not developed at birth and babies are born with mechanisms of cry to convey their discomfort and call for attention. The vocalization pattern in cry varies, but it is hard to differentiate the cry types. Pediatricians follow the Wessel rule of 3 for colic-related excessive cries, according to which infantile colic is defined as inconsolable crying for 3 hours per day at least three times a week for at least 3 weeks. But still in the majority of infants, this compliance is not observed. In a few recent studies, attempts were made to label the cry types made in normal physiological state and various conditions like malnutrition, preterm birth, hypoxia, pneumonia, sudden infant death syndrome, laryngomalacia, etc.24,25,26,27,28,29,30,31,32,33 It is also noted that voice recognition is based on individual-specific voice traits like finger prints as the individual differences in human voice pitch are preserved.34
Ultrasonic Vocalizations Pattern to Assess the Emotional Development in Pups from Postnatal Days 1 to 21
Rodents also communicate through ultrasonic vocalizations (USVs) in the range of 20 to 100 kHz, which are inaudible to human ears in addition to making a few audible calls during any painful handling. After birth, the rat dam (mother) keeps the pups huddled for maintaining their body temperature, nursing, and for frequent grooming (fecal removal training, somatosensory stimulation). USVs are recorded using special microphones in the isolation paradigm wherein pups are taken away from their mother for 2 minutes in a soundproof chamber.8,9,16 Pups born to either REM sleep-restricted (22 h/d, 11 a.m. to 9 a.m. from gestational day 15 to 20) mothers (rat dams) or total sleep-restricted (5 h/d, 9 a.m. to 2 p.m., from gestational day 15 to 20) mothers, from PND 1 to 21, showed a distinct pattern of USVs in terms of both quantity and quality (►Fig. 3). In control pups, the number of USVs is low after birth, but it begins to increase gradually peaking on PND 9 followed by a decrease until PND 21. Compared to controls, pups born to the REM sleep-restricted mother not only made lesser calls on the PNDs 1 to 11 but also showed development delay in vocalization patterns.8 However, the pups born to total sleep-restricted dams cried more compared to controls on initial PNDs 1 to 9 that appeared reaching to normalcy on later days.9 Normal pups show a specific pattern of call types, making simpler constant frequency calls during initial PNDs. They gradually gained the ability to produce frequency modulation with progression of age.16 Moreover, USVs reduced to minimal in normal pups (C) around the PND 21 when separation from the mother is no more a stress to them as they become more independent in their habits, and depending less on mothers for their needs. But pups born to total sleep-restricted dams made a higher pitch (increased bandwidth) in upward call type and also in the two-component step calls, which help in seeking more maternal attention (►Fig. 3). This is similar to abnormal calling patterns reported in pups born to mothers undergoing malnutrition during the prenatal period.35

- Qualitative and quantitative pattern in ultrasonic vocalizations (USVs) in pups during various postnatal days born to control and rapid eye movement (REM) restricted rat dams. (A) Developmental patterns in USVs in pups born to control and REM restricted dams from postnatal days 1 to 21. (B) Higher intensity in call type (2 syllable) in pups from the REM sleep restriction (REMSR) dams. (C) Various categories of USVs. (D) Changes in call types in pups on days 1, 11, and 21 in control and the REMSR group. (Adapted from Gulia et al.8)
Possibly the altered USVs in the absence of optimal nest environment and maternal care during early postnatal period can signal the emotional deficits during later life. The exact biological communication hidden in the individual call types are not yet deciphered, but it would be interesting to decode these acoustic signals to understand the nature of these deficits. Reverse communication also exists and pup vocalizations do influence the mother's behavior as the USVs of the pups shape the mother's responsiveness toward the pups.36 Alterations in USVs in pups could be due to prenatal factors alone or with a contribution from postnatal factors. However, it seems that the brain development during early postnatal period is important in determining cognitive functions37 and also impaired neural development during fetal life may increase the susceptibility to emotional disturbances.21,38
Effects of Sleep Loss during Pregnancy on Sleep–Wakefulness during Early Development in Offspring
In altricial species including humans, babies are born immature. The fetal brain undergoes fast development during the third trimester of pregnancy that continues to develop until the adolescent period to achieve optimal cognitive abilities for survival. Fetus sleep exists in a primitive form consisting of the active sleep (AS; precursor of REM sleep) and the quiet sleep (QS; precursor of NREM sleep). Term-born human neonates spend about 80 to 85% of time in sleep, of which nearly 50% of the component is AS. With development, the percentage of AS gradually keeps on decreasing and reaches about 20 to 25% in young adults. Sleep pattern is also polyphasic wherein babies wake up regularly across 24 hours, as circadian rhythms are not yet developed.39
It is a challenging task to study newborn rat pups owing to their tiny size and unossified skull, lack of thermoregulatory abilities, and closed eyes/ears at birth. For studying their S-W, acute implantation of EEG and EMG electrodes was carried out, and pups were studied in a special fabricated environmental chamber in which temperature and humidity are regulated.13,14,15 Similar to human neonates, rat pups also show relatively shorter bouts of sleep episodes compared to adults. In these newborn rats, AS constitutes a substantial period of time (about 70–75%) and they spent comparatively lesser time in QS (about 10%). This pattern is observed during the initial week and thereafter the AS reduces, while the percentage of QS rises. After 10 days, AS decreases dramatically, while QS increases exponentially. Also, the bouts of AS become shorter, while the length of QS increases. A higher percentage of AS is found in immature newborns albeit there are species differences.13 Behavioral events in rats during AS in the initial PNDs 1 to 7 include rapidly occurring myoclonic twitches and jerky limb movements. Myoclonic twitches and subsequent hippocampal neocortical communication probably assist in the development of cortical neural networks and somatosensory responses.40,41
Sleep restriction during the last trimester of pregnancy provided insights into brain plasticity for sleep networks in growing neonates. REMSR or TSR during the third trimester of pregnancy not only affected the natural ontogenetic development of sleep in utero but also delayed and disrupted the normal development of S-W.13,14,17 Compared to age-matched control, pups from both groups of sleep-restricted dams displayed a higher percentage of AS and a lesser percentage in QS. Longer duration of the S-W cycles in neonates and infants of sleep-restricted mothers indicated vulnerability of this parameter during fetal development. Reduced S-W cycles are also observed in preterms (younger than 30 weeks of gestational age) as compared to the control group.42 The circadian rhythms in these preterm neonates are also less robust as they exhibit a multitude of ultradian frequencies, while full-term neonates show a distinct circadian frequency depicting fast initial adaptation in the first week of life to a 24-hour day.43,44 In altricial species where babies are born immature (e.g., human babies born between 27 and 37 weeks), AS is high at the time of birth.45,46 On the contrary, full-term pups of sleep-restricted dams also display immature patterns of sleep at the time of birth.13,14 This clearly indicates that maternal sleep restriction during the last term of pregnancy delays the maturation of S-W in utero. This may not be due to maternal stress during sleep restriction, as infants of mothers who consumed alcohol showed reduction in the AS and increase in wakefulness.47,48 Mothers exposed to cigarette smoke during pregnancy (passive smoke) had babies with impaired arousal patterns and were more susceptible to sudden infant death syndrome.49,50
Altered Sleep Microarchitecture during Development
In the developing brain, the EEG power spectral analysis is an established method to understand the power distribution in different EEG frequency bands. Such analysis performed during postnatal days 15 (D15) and 20 (D20) during QS and AS sleep provided an interesting insight into the development of these bands.17 In control pups, during QS, delta power decreases from D15 to D20, whereas powers in theta, alpha, and beta bands increase. However, pups born to REMSR dams exhibited lower delta power than controls on D15, while there was high power on other frequencies (beta and theta) in the QS traces. Besides this, the delta power did not decrease during D20 but remained higher, while the alpha, beta, and theta powers decreased significantly. During AS, there was higher delta power, but lesser theta and beta powers on D15. Higher power in the theta band and lower powers in alpha and beta on D20 indicated imbalance and delayed development of networks involved in EEG. Similar trends are observed in preterm fetal growth restricted neonates.51 Alterations in thalamocortical cortical network organization can reduce the percentage of delta power. Decreased delta powers during slow wave sleep are also evident in depressed patients52 with increased high-frequency activity,53 which may be considered as markers of a susceptibility to stress and psychiatric disorders. Compared to full-term newborns, the premature group exhibited a higher frequency and larger amplitude of beta activity and also better inter- and intrahemispheric relationships. In human neonates, high-density EEG (noninvasive) can be used to examine brain maturation in infants who are at risk of neurodevelopmental deficits.
Clues from Modeling of Distribution Patterns of S-W Bouts during Development
Mathematical models are used to predict the precise developmental accounts of the neural foundations of sleep.54 In human adults, sleep bouts follow an exponential distribution, whereas wake bouts follow a power law distribution and similar patterns are true in adult rats, cats, and mice.54 Exponential processes imply probabilistic state changes typically governed by a constant rate of change over time. As sleep follows the same exponential dynamics, the distribution of S-W transitions may thus hold important clues to understanding the mechanisms underlying sleep architecture in health and disease.55 Power law distributions arise from multiple components of a system interacting in a complex manner and often follow a similar profile across multiple measurement scales.
We also tested the generalizability of the S-W bout distribution from PNDs 1 to 20 in pups born to total sleep-restricted dams. The duration of sleep bouts exhibited exponential distributions similar to previous reports.13,54 Decreased slope in the log-survival plots for bout durations of sleep in the TSR group on all the studied PNDs not only featured a delay in the development of sleep networks but also depicted the vulnerability of sleep maturation process. Strikingly, due to underdeveloped wake networks during early PNDs 1 to 10, the survival plots for wakefulness were least altered. However, during PNDs 15 and 20, compromised wakefulness distribution suggested that a sleep bout is a stochastic process having a steady probability of transition into wakefulness, which decreases through development, thereby achieving longer episodes of sleep. Wake bouts in very young rats also follow an exponential distribution during initial development (PNDs 1–10), but the distribution of wake bouts followed the power law from PND 15 onward. The probability distribution during the third week of development indicated that an already lengthy wake bout is likely to persist a little longer. Sleep and wake bout lengths show no memory for the duration of any previous episode, so that transitions between these states resemble an alternating renewal process.56 These modeling studies were useful in understanding the development of sleep architecture, fragmentation, and associated network formations.
Detection of sleep changes in neonates may be of great clinical significance and can be viewed as early markers of psychobehavioral disorders. Decreased QS during early PNDs (1–10) and comparatively increased AS during all postnatal developmental days in the offspring of REM sleep-restricted mothers reflect downregulation of the maturational process. Pups of REM sleep-restricted mothers had reduced REM sleep latency during PNDs 15 to 20 that are indicative of depressionlike traits. Early onset of REM sleep is commonly observed in the subjects with depression and narcolepsy. Moreover, the impaired sleep regulation including altered sleep homeostasis, reduced REM latency, reduced slow-wave sleep, and increased REM duration are commonly observed in depressed patients.
Possible Disruption of Neural Networks for S-W in Newborns after Maternal Sleep Restriction
In general, the AS mechanisms located in the brainstem mature first and the brain mechanisms for QS expression develop comparatively later. But QS is also affected by maternal sleep restriction. The reduced QS percentage in the pups of REM sleep-restricted dams indicated suppression of QS organization. Furthermore, the persistence of higher level of AS/REM sleep until PND 20 probably indicated the existence of the immature brain lacking inhibitory control over the AS/REM sleep.6 Greater amount of QS observed in infants of depressed mothers is considered a conservation–withdrawal phenomenon since information processing for both auditory and visual stimulation is altered in depressed individuals during deep sleep. In addition, decreased wakefulness also indicate a delay in natural development of wake-promoting regions in the brain, including the locus coeruleus, which contributes to the developmental transition in the S-W pattern.
Circadian rhythms for S-W develop at around 2 months of age in humans. Since melatonin is one important hormone for sleep regulation, maintenance of an optimal maternal circadian rhythmicity via the suprachiasmatic nuclei would be essential to program the developing master oscillator of the fetus melatonin cycle. Chronodisruption and disturbed melatonin cycles have a negative impact on the maturing fetal oscillators, which may contribute to psychological and behavioral problems in the offspring. Preterm infants that may be deprived of some exposure to a normal maternal melatonin cycle show delayed development of the circadian rhythm.
Changes in the Behavioral Traits of the Pups (Emotional Testing in Elevated Plus Maze)
At the time of birth, the testing of UVs is the suitable method to understand their emotional profile. However, postweaning, the emotional behavior during the periadolescent window care carried out through elevated plus maze (EPM) with a video-tracking system. As the name suggests, a maze in shape of plus sign is kept elevated from the surface of the floor. Two arms of the plus maze are open, while the remaining two are closed ones. Rodents, being burrowing animals, like to spend more time in the closed chamber as compared to the open arms. Various parameters of the EPM test conducted individually for each rat (5 minutes) provide their emotional status.10 The pups born to the TSR dam showed distinct hyperactivity during the periadolescent period.10 They also enhanced novelty-seeking and risk-taking behavior. These findings suggest that maternal sleep loss during the prenatal period might result in emotional instability and behavioral deficits in pups during postnatal life. Weanlings from the REMSR group did not show such changes. However, these rats (REMSR group) showed increased immobility time and decreased latency to swim in the forced swim test, indicating persistence of depressionlike symptoms.
Drastic reduction in the USVs until PND 11 and higher calling rate during PND 21 are the earliest signal of behavioral alterations in REMSR rats. When these rats grew up to the adult stage, they showed deficits in emission of mating vocalizations (data from our unpublished findings) that mark persistence of long-term effects in the offspring from the REMSR mothers.
Sleep Deprivation–Induced Learning and Memory Impairment in Adults (Rats)
Sleep deprivation–induced impairment in learning and memory in adult rats are manifested through altered functional connectivity among the hippocampus, amygdala, and prefrontal cortex during REM sleep.17 In these set of experiments, in addition to EEG and EMG electrodes for recording S-W, microelectrodes were stereotactically implanted deep into the brain (pons, amygdala, and prefrontal cortex).17 Their REM sleep is characterized by the presence of theta waves and P waves (in rats) similar to the ponto-geniculo-occipital (PGO) waves in humans, in addition to desynchronized EEG and muscle atonia, in addition to several other physiological changes.57,58 The PGO waves originate from the pontine area and spread to the geniculate and occipital areas and are associated with learning and memory. Total sleep deprivation of 24 hours (both components of sleep) elicited changes in the P waves, and theta coherence among the hippocampus, amygdala, and the prefrontal cortex, affecting the reference and working memory in rats.12 This study emphasized the crucial role of sleep in maintaining an appropriate synchrony between various neuronal networks during REM sleep. It is comparatively easier to conduct such studies in adults, but it is always very challenging in neonates and infants.
It is getting well established now that prenatal disruptions in sleep patterns can give rise to various neuropsychiatric disorders in the offspring. Sleep can be seen as an epiphenomenon for neurocognitive network activities as any acute and chronic sleep disruptions can influence brain activity, resulting in impaired behavior.17,59 Sleep deprivation during pregnancy suppressed the hippocampal long-term potentiation in rat offsprings.60 All these studies strongly indicate the role of prenatal sleep in fetal programming.61 Moreover, recent reviews strengthen the prenatal origin of neuropsychiatric diseases especially depression and anxiety.15,17,18,62,63,64,65 More genome-wide association studies are required to tease out the effects of prenatal and postnatal contribution in neuropsychiatric diseases in humans.66
Sleep loss during pregnancy is a potential risk factor for various psychological disorders in the offspring, which is also emphasized through a few recent meta-analyses.10,16,67,68,69 These findings from the rat model illustrate the importance of adequate sleep during late pregnancy for proper neuropsychological development in the offspring (►Fig. 4). Lack of NREM sleep during late pregnancy can lead to the development of hyperactivity and increased risk-taking behavior in the offspring. REM sleep deprivation during pregnancy can lead to depressionlike symptoms in babies and deficits in sexual behavior in later life due to impaired USV communication during mating. The neuropeptide orexin contributes in shaping an appropriate arousal system.70 It is to be noted that spontaneous penile erections (in males) and clitoris engorgements (in females) are observed during REM sleep irrespective of age. Our group have provided evidences for involvement of the septal neurons in these sleep-related erections in adults, indicating a wider role of sleep in organizing the vital functions of the body.71,72,73,74 Further, knocking the peripheral warm receptors postnatally, by injecting capsaicin in neonatal rats, the central warm receptors produced adaptive changes to defend the high ambient temperature by increasing NREM sleep in adults.75 It will be interesting to explore in future studies if prenatal sleep disruption also influences erectile and thermoregulatory networks. All these elegant studies unearth the importance of sleep during late pregnancy for a healthy brain programming and functioning. Moreover, further electrophysiological, molecular, and genetic studies in neonatal life would help in understanding complex interactions of several neural networks in brain as sleep, thermoregulation, sexual functions, and behavioral regulation are overlapping.76,77,78,79,80

- Summary of effects of sleep restriction on various parameters across different postnatal days (PND). AS, active sleep; QS, quiet sleep; SW, sleep–wakefulness; USVs, ultrasonic vocalizations.
Conclusion
Evidences from both the controlled studies carried out in rodent models and the epidemiological studies in human subjects emphasized on the fact that decreased sleep quality during pregnancy is a potential novel risk factor for neurocognitive development of the offspring.4,5,6,18,67,68,69,76 It is a challenging task to take optimal care of newborns with a relatively immature brain. There is pestering need to conduct research in humans as well as animal models to explore the effects of unavoidable photic and other stimulations in the neonatal ICUs (the preterm human babies) on the development of an optimal arousal system as these critical conditions of postnatal hyperstimulation may affect autonomic nervous and alter behavior in growing babies. On the other hand, underdiagnosed term born babies with an immature brain (difficult to diagnose without EEG parameters) pose another challenge. Extensive meticulous research is needed to study the effects of disruptions of postnatal sleep in babies on the developmental timelines due to vulnerability of networks for S-W, autonomic nervous system, and motor and somatosensory networks in relation to onset of neuropsychiatric disease. It is already pointed out that various prenatal factors including environmental and psychosocial stress are detrimental to the fetal developmental programming, which is reflected during later developmental trajectories of life.72,73,74,75 Since the newborn requires maternal care for a reasonably long time in humans, it is essential to tease out the role of sleep and mechanisms for developmental disruption of the brain in utero and during postpartum growth. Further research is needed to understand the role of sleep in the pre- and postnatal genesis of neuropsychiatric diseases. Also, since the process of the development of the brain is so complex, preventive measures must be implemented through formulation and implementation of the strong public health policies. A cry detector innovative app based on artificial intelligence may be developed to detect the early development abnormality in human neonates as a noninvasive tool. It is high time that we give priority to good-quality sleep during pregnancy and also during the remaining phases of life in the current era for a healthy life and well-being.
Acknowledgment
KKG expresses special acknowledgement to Prof. V. Mohan Kumar for his support and also for being the Co-Investigators in these studies. KKG acknowledges the National Academy of Medical Sciences (NAMS), New Delhi, for conferring the Dr. B.K. Anand Oration Award 2020–2021 to her and for providing the opportunity to write this review as a summary of the oration lecture.
Conflict of Interest
None declared.
Funding
These studieswere supported by research grants fromthe Cognitive Science Research Initiation Program of Department of Science and Technology, India (SR/CSI/110/2012 and SR/CSRI/102/2014).
References
- Regularly occurring periods of eye motility, and concomitant phenomena, during sleep. Science. 1953;118(3062):273-274.
- [CrossRef] [PubMed] [Google Scholar]
- Control of sleep and wakefulness. Physiol Rev. 2012;92(03):1087-1187.
- [CrossRef] [PubMed] [Google Scholar]
- How disturbed sleep may be a risk factor for adverse pregnancy outcomes. Obstet Gynecol Surv. 2009;64(04):273-280.
- [CrossRef] [PubMed] [Google Scholar]
- Sleep deprivation during pregnancy and maternal and fetal outcomes: is there a relationship? Sleep Med Rev. 2010;14(02):107-114.
- [CrossRef] [PubMed] [Google Scholar]
- Sleep impairment during pregnancy: possible implications on mother-infant relationship. Med Hypotheses. 2010;75(06):578-582.
- [CrossRef] [PubMed] [Google Scholar]
- Reduction in ultrasonic vocalizations in pups born to rapid eye movement sleep restricted mothers in rat model. PLoS One. 2014;9(01):e84948.
- [CrossRef] [PubMed] [Google Scholar]
- Increased ultrasonic vocalizations and risk-taking in rat pups of sleep-deprived dams. Physiol Behav. 2015;139:59-66.
- [CrossRef] [PubMed] [Google Scholar]
- Sleep deprivation during late pregnancy produces hyperactivity and increased risk-taking behavior in offspring. Brain Res. 2015;1596:88-98.
- [CrossRef] [PubMed] [Google Scholar]
- Dynamic changes in sleep pattern during post-partum in normal pregnancy in rat model. Behav Brain Res. 2017;320:264-274.
- [CrossRef] [PubMed] [Google Scholar]
- Ultrasonic vocalizations and behavior of rat pups born from sleep-deprived dams. In: Brudzynski SM. ed. Handbook of Behavioral Neuroscience Series. Handbook of Ultrasonic Vocalizations: A Window into the Emotional Brain. Vol. 25. Philadelphia, PA: Elsevier; 2018:467-478.
- [CrossRef] [Google Scholar]
- The effects of rapid eye movement sleep deprivation during late pregnancy on newborns' sleep. J Sleep Res. 2018;27(02):197-205.
- [CrossRef] [PubMed] [Google Scholar]
- Immature sleep pattern in newborn rats when dams encountered sleep restriction during pregnancy. Int J Dev Neurosci. 2018;69:60-67.
- [CrossRef] [PubMed] [Google Scholar]
- Developmental aspects of sleep. In: Gozal D, Kheirandish-Gozal L. eds. Pediatric Sleep Medicine. Cham: Springer; 2021:115-122.
- [CrossRef] [Google Scholar]
- Reduced theta coherence and P wave ratio linked to memory deficits after sleep deprivation in rat model. Sleep Vigil. 2017;1:21-29.
- [CrossRef] [Google Scholar]
- Proper sleep during pregnancy for mental health of new-born: an evidence based appeal to policy makers. Sleep Vigil. 2018;2:97-98.
- [CrossRef] [Google Scholar]
- Effects of sleep modulation during pregnancy in the mother and offspring: evidence from preclinical research. J Sleep Res. 2021;30(03):e13135.
- [CrossRef] [PubMed] [Google Scholar]
- Infant cry: a clue to diagnosis. Pediatrics. 1982;69(02):197-201.
- [CrossRef] [PubMed] [Google Scholar]
- Development of translational methods in spectral analysis of human infant crying and rat pup ultrasonic vocalizations for early neurobehavioral assessment. Front Psychiatry. 2011;2:56.
- [CrossRef] [PubMed] [Google Scholar]
- Prenatal stress and neurodevelopment of the child: focus on the HPA axis and role of the placenta. Dev Neurosci. 2009;31(04):285-292.
- [CrossRef] [PubMed] [Google Scholar]
- Developmental outcome prediction from acoustic cry analysis in term and preterm infants. Pediatrics. 1987;80(04):529-534.
- [CrossRef] [PubMed] [Google Scholar]
- Assessment of infant cry: acoustic cry analysis and parental perception. Ment Retard Dev Disabil Res Rev. 2005;11(01):83-93.
- [CrossRef] [PubMed] [Google Scholar]
- Newborn acoustic cry characteristics of infants subsequently dying of sudden infant death syndrome. Pediatrics. 1995;96(1, Pt 1):73-77.
- [CrossRef] [PubMed] [Google Scholar]
- Cry analysis in infants with severe malnutrition. Eur J Pediatr. 1978;128(04):241-246.
- [CrossRef] [PubMed] [Google Scholar]
- Translational analysis of effects of prenatal cocaine exposure on human infant cries and rat pup ultrasonic vocalizations. PLoS One. 2014;9(10):e110349.
- [CrossRef] [PubMed] [Google Scholar]
- Data collection of infant cries for research and analysis. J Voice. 2017;31(02):252.e15-252.e26.
- [CrossRef] [PubMed] [Google Scholar]
- Application of pattern recognition techniques to the classification of full-term and preterm infant cry. J Voice. 2016;30(06):656-663.
- [CrossRef] [PubMed] [Google Scholar]
- Cry-based infant pathology classification using GMMs. Speech Commun. 2016;77:28-52.
- [CrossRef] [PubMed] [Google Scholar]
- A database of infant cry sounds to study the likely causes of cry. Paper presented at: Proceedings of the 12th International Conference on Natural Language Processing; December 11–14 2015 Trivandrum, India
- [Google Scholar]
- Identification of diseases in newborns using advanced acoustic features of cry signals. Biomed Signal Process Control. 2019;50:35-44.
- [CrossRef] [PubMed] [Google Scholar]
- Acoustic features of cry of infants with high risk factors. Int J Health Sci Res. 2019;9(12):62-67.
- [Google Scholar]
- Defining and distinguishing infant behavioral states using acoustic cry analysis: is colic painful? Pediatr Res. 2020;87(03):576-580.
- [CrossRef] [PubMed] [Google Scholar]
- Individual differences in human voice pitch are preserved from speech to screams, roars and pain cries. R Soc Open Sci. 2020;7(02):191642.
- [CrossRef] [PubMed] [Google Scholar]
- Ultrasonic call characteristics of rat pups are altered following prenatal malnutrition. Dev Psychobiol. 2003;43(02):90-101.
- [CrossRef] [PubMed] [Google Scholar]
- Pups call, mothers rush: does maternal responsiveness affect the amount of ultrasonic vocalizations in mouse pups? Behav Genet. 2005;35(01):103-112.
- [CrossRef] [PubMed] [Google Scholar]
- Birth weight and later risk of depression in a national birth cohort. Br J Psychiatry. 2004;184:28-33.
- [CrossRef] [PubMed] [Google Scholar]
- Critical periods of brain growth and cognitive function in children. Brain. 2004;127(Pt 2):321-329.
- [CrossRef] [PubMed] [Google Scholar]
- Sleep disorders in the elderly: a growing challenge. Psychogeriatrics. 2018;18(03):155-165.
- [CrossRef] [PubMed] [Google Scholar]
- The union of the state: myoclonic twitching is coupled with nuchal muscle atonia in infant rats. Behav Neurosci. 2002;116(05):912-917.
- [CrossRef] [PubMed] [Google Scholar]
- Benign neonatal sleep myoclonus evokes somatosensory responses. J Clin Neurophysiol. 2017;34(06):484-491.
- [CrossRef] [PubMed] [Google Scholar]
- Background patterns and sleep-wake cycles on amplitude-integrated electroencephalography in preterms younger than 30 weeks gestational age with peri-/intraventricular haemorrhage. Acta Paediatr. 2007;96(12):1743-1750.
- [CrossRef] [PubMed] [Google Scholar]
- Ultradian and circadian activity-rest rhythms of preterm neonates compared to full-term neonates using actigraphic monitoring. Chronobiol Int. 2001;18(04):697-708.
- [CrossRef] [PubMed] [Google Scholar]
- Development of sleep-wakefulness rhythm in premature babies. Acta Paediatr. 1995;84(05):484-489.
- [CrossRef] [PubMed] [Google Scholar]
- The development of sleeping and waking states in high-risk preterm infants. Infant Behav Dev. 1990;13:513-531.
- [CrossRef] [Google Scholar]
- Preterm infant state development. J Obstet Gynecol Neonatal Nurs. 2008;37:657-665. PubMed
- [CrossRef] [PubMed] [Google Scholar]
- Sleep disturbances after acute exposure to alcohol in mothers' milk. Alcohol Fayettev N. 2001;25:153-158. PubMed
- [CrossRef] [PubMed] [Google Scholar]
- Effects of prenatal alcohol exposure on neonatal sleep-wake behaviour and adult alcohol consumption in rats. Acta Pharmacol Toxicol (Copenh). 1986;59(01):36-42.
- [CrossRef] [PubMed] [Google Scholar]
- Smoking during pregnancy: postnatal effects on arousal and attentional brain systems. Neurotoxicology. 2007;28(05):915-923.
- [CrossRef] [PubMed] [Google Scholar]
- Maternal smoking impairs arousal patterns in sleeping infants. Sleep. 2009;32(04):515-521.
- [CrossRef] [PubMed] [Google Scholar]
- EEG power spectrum maturation in preterm fetal growth restricted infants. Brain Res. 2018;1678:180-186.
- [CrossRef] [PubMed] [Google Scholar]
- The S-deficiency hypothesis of depression and the two-process model of sleep regulation. Pharmacopsychiatry. 1987;20(01):23-29.
- [CrossRef] [PubMed] [Google Scholar]
- Delta, theta, alpha and beta power spectrum of sleep electroencephalogram in infants aged two to eleven months. Sleep. 1983;6(04):376-383.
- [CrossRef] [PubMed] [Google Scholar]
- Common scale-invariant patterns of sleep-wake transitions across mammalian species. Proc Natl Acad Sci U S A. 2004;101(50):17545-17548.
- [CrossRef] [PubMed] [Google Scholar]
- Power law versus exponential state transition dynamics: application to sleep-wake architecture. PLoS One. 2010;5(12):e14204.
- [CrossRef] [PubMed] [Google Scholar]
- Dynamics of sleep-wake cyclicity in developing rats. Proc Natl Acad Sci U S A. 2005;102(41):14860-14864.
- [CrossRef] [PubMed] [Google Scholar]
- Neuronal activity in the caudolateral peribrachial pons: relationship to PGO waves and rapid eye movements. J Neurophysiol. 1994;71(01):95-109.
- [CrossRef] [PubMed] [Google Scholar]
- Brainstem-diencephalo-septohippocampal systems controlling the theta rhythm of the hippocampus. Neuroscience. 1997;81(04):893-926.
- [CrossRef] [PubMed] [Google Scholar]
- Dynamism in activity of the neural networks in brain is the basis of sleep-wakefulness oscillations. Front Neurol. 2012;3:38.
- [CrossRef] [PubMed] [Google Scholar]
- Fetal programming of coronary heart disease. Trends Endocrinol Metab. 2002;13(09):364-368.
- [CrossRef] [PubMed] [Google Scholar]
- Prenatal origins of neuropsychiatric diseases. Acta Paediatr. 2021;110(06):1741-1749.
- [CrossRef] [PubMed] [Google Scholar]
- Fetal origins of adult disease. Curr Probl Pediatr Adolesc Health Care. 2011;41(06):158-176.
- [CrossRef] [PubMed] [Google Scholar]
- Prenatal developmental origins of future psychopathology: mechanisms and pathways. Annu Rev Clin Psychol. 2019;15:317-344.
- [CrossRef] [PubMed] [Google Scholar]
- Research review: developmental origins of depression—a systematic review and meta-analysis. J Child Psychol Psychiatry. 2021;62(09):1050-1066.
- [CrossRef] [PubMed] [Google Scholar]
- Maternal sleep deprivation at different stages of pregnancy impairs the emotional and cognitive functions, and suppresses hippocampal long-term potentiation in the offspring rats. Mol Brain. 2016;9:17.
- [CrossRef] [PubMed] [Google Scholar]
- Distinct effect of prenatal and postnatal brain expression across 20 brain disorders and anthropometric social traits: a systematic study of spatiotemporal modularity. Brief Bioinform. 2021;22(06):bbab214.
- [CrossRef] [PubMed] [Google Scholar]
- Maternal sleep during pregnancy and poor fetal outcomes: a scoping review of the literature with meta-analysis. Sleep Medicine Reviews. 2018;41:197-219. PubMed
- [CrossRef] [PubMed] [Google Scholar]
- Maternal sleep during pregnancy and adverse pregnancy outcomes: a systematic review and meta-analysis. J Diabetes Investig. 2022;13(07):1262-1276.
- [CrossRef] [PubMed] [Google Scholar]
- Association between adverse perinatal outcomes and sleep disturbances during pregnancy: a systematic review and meta-analysis. J Matern Fetal Neonatal Med. 2022;35(01):166-174.
- [CrossRef] [PubMed] [Google Scholar]
- Orexin A (hypocretin-1) application at the medial preoptic area potentiates male sexual behavior in rats. Neuroscience. 2003;116(04):921-923.
- [CrossRef] [PubMed] [Google Scholar]
- Sleep-related penile erections do not occur in rats during carbachol-induced rapid eye movement sleep. Behav Brain Res. 2004;154(02):585-587.
- [CrossRef] [PubMed] [Google Scholar]
- Role of the lateral septal noradrenergic system in the elaboration of male sexual behavior in rats. Pharmacol Biochem Behav. 2002;72(04):817-823.
- [CrossRef] [PubMed] [Google Scholar]
- The septal area, site for the central regulation of penile erection during waking and rapid eye movement sleep in rats: a stimulation study. Neuroscience. 2008;156(04):1064-1073.
- [CrossRef] [PubMed] [Google Scholar]
- Assessment of the septal area neuronal activity during penile erections in rapid eye movement sleep and waking in the rats. J Physiol Sci. 2018;68(05):567-577.
- [CrossRef] [PubMed] [Google Scholar]
- Ambient temperature related sleep changes in rats neonatally treated with capsaicin. Physiol Behav. 2005;85(04):414-418.
- [CrossRef] [PubMed] [Google Scholar]
- Sleep disorder diagnosis during pregnancy and risk of preterm birth. Obstet Gynecol. 2017;130(03):573-581.
- [CrossRef] [PubMed] [Google Scholar]
- Fetal origins of mental health: the developmental origins of health and disease hypothesis. Am J Psychiatry. 2017;174(04):319-328.
- [CrossRef] [PubMed] [Google Scholar]
- Prenatal stress and genetic risk: how prenatal stress interacts with genetics to alter risk for psychiatric illness. Psychoneuroendocrinology. 2018;90:9-21.
- [CrossRef] [PubMed] [Google Scholar]
- Maternal sleep quality during pregnancy is associated with neonatal auditory ERPs. Sci Rep. 2020;10(01):7228.
- [CrossRef] [PubMed] [Google Scholar]
- Gestational intermittent hypoxia induces endothelial dysfunction, reduces perivascular adiponectin and causes epigenetic changes in adult male offspring. J Physiol. 2019;597(22):5349-5364.
- [CrossRef] [PubMed] [Google Scholar]